Learning Objectives
For the AP Chemistry exam, you should understand the definition and function of catalysts in lowering activation energy and speeding up reactions. Differentiate between homogeneous and heterogeneous catalysts, providing examples of each, and grasp the role of enzymes as biological catalysts with concepts like substrate specificity and active sites. Interpret energy diagrams showing catalyzed versus uncatalyzed reactions, and analyze how catalysts affect rate laws and reaction mechanisms. Recognize the industrial and environmental applications of catalysts, such as in the Haber process and catalytic converters. Develop problem-solving skills to apply these concepts in practice exams, and familiarize yourself with experimental techniques for studying catalytic effects.
Introduction
Catalysis is a crucial concept in chemistry where a substance called a catalyst speeds up a chemical reaction without being consumed in the process. This means that even small amounts of a catalyst can significantly increase the reaction rate, making processes more efficient. Catalysts are vital in various industries, from manufacturing to pharmaceuticals, as they help produce desired products faster and with less energy. Understanding how catalysts work involves exploring the ways they lower the activation energy needed for reactions, enabling molecules to react more easily.
What is Catalysis?
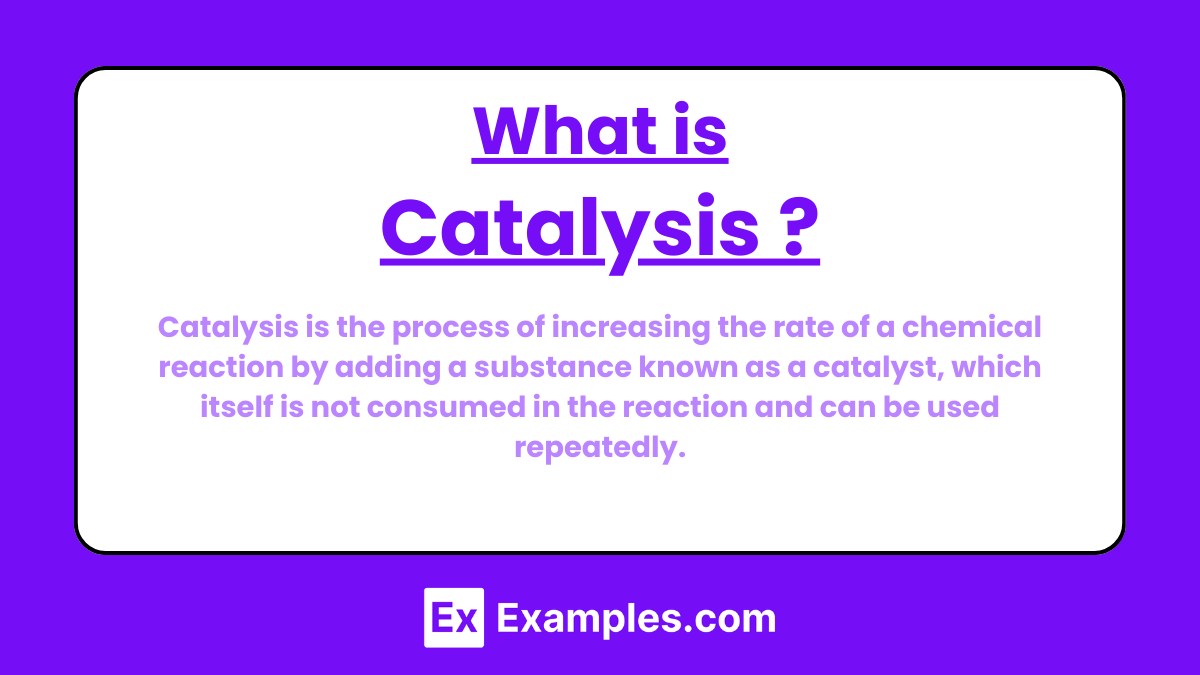
Catalysis is the process of increasing the rate of a chemical reaction by adding a substance known as a catalyst, which itself is not consumed in the reaction and can be used repeatedly. The catalyst provides an alternative reaction pathway with a lower activation energy, thus enabling the reaction to proceed more quickly or under milder conditions.
How Catalysts Work
Catalysts work by providing an alternative reaction pathway with a lower activation energy. This lower energy pathway allows more reactant molecules to have enough energy to react, increasing the reaction rate without the catalyst being consumed in the process.
Mechanism:
- Adsorption (for Heterogeneous Catalysts): Reactant molecules adsorb onto the catalyst’s surface, weakening bonds and lowering activation energy.
- Intermediate Formation: The catalyst interacts with reactants to form a lower-energy intermediate complex.
- Reaction and Desorption: The intermediate decomposes to form the products, which then desorb from the catalyst, leaving it free to catalyze another reaction.
Energy Diagram:
- Uncatalyzed Reaction: Higher activation energy peak.
- Catalyzed Reaction: Lower activation energy peak, indicating an alternative pathway.
Types of Catalysts
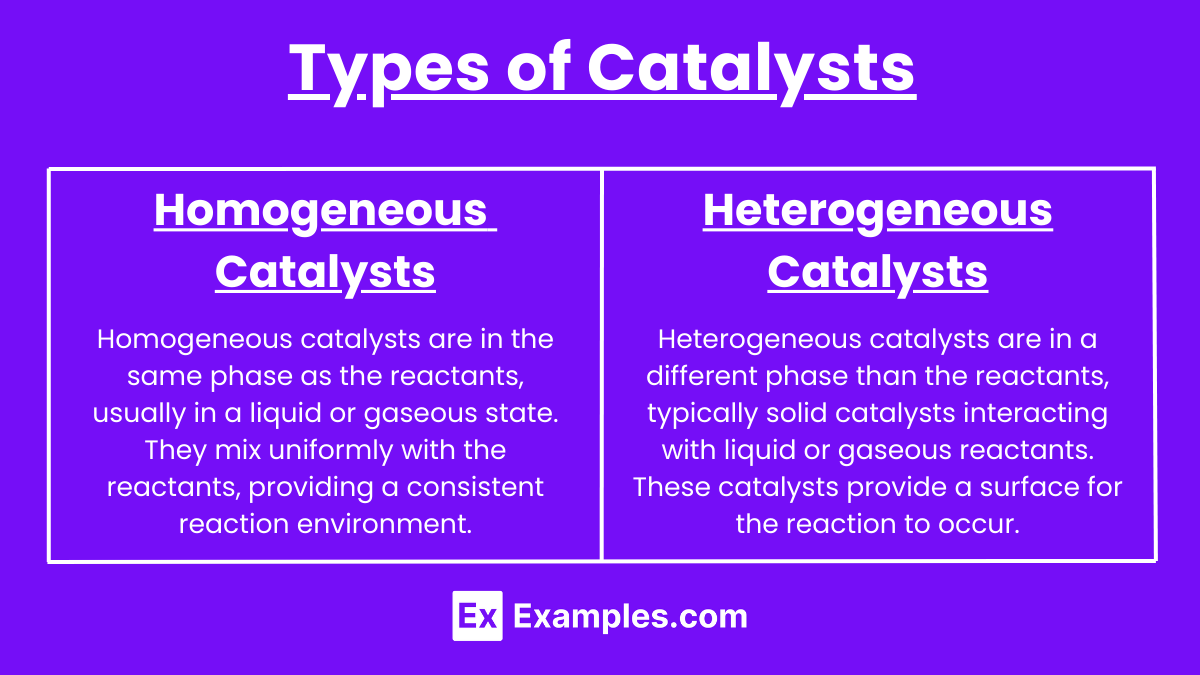
Catalysts can be broadly classified into two main types: homogeneous catalysts and heterogeneous catalysts. Each type has distinct characteristics and applications.
Homogeneous Catalysts
Homogeneous catalysts are in the same phase as the reactants, usually in a liquid or gaseous state. They mix uniformly with the reactants, providing a consistent reaction environment.
Key Characteristics:
- Phase Consistency: Homogeneous catalysts are in the same phase as the reactants, ensuring uniform interaction.
- Reaction Conditions: Often used in reactions that occur in solution, such as liquid-liquid or gas-gas reactions.
- Ease of Study: Since they mix completely with the reactants, it’s easier to study their mechanisms.
Examples:
- Acid Catalysis: Sulfuric acid (H₂SO₄) catalyzes the esterification of alcohols.
- Base Catalysis: Sodium hydroxide (NaOH) catalyzes the hydrolysis of esters.
- Transition Metal Complexes: Palladium (Pd) complexes are used in homogeneous catalytic processes like the Heck reaction.
Heterogeneous Catalysts
Heterogeneous catalysts are in a different phase than the reactants, typically solid catalysts interacting with liquid or gaseous reactants. These catalysts provide a surface for the reaction to occur.
Key Characteristics:
- Phase Difference: Heterogeneous catalysts are usually solids, while the reactants are in liquid or gas phases.
- Surface Interaction: The reaction occurs on the surface of the catalyst, which provides active sites for the reactants.
- Separation and Reuse: Easier to separate from the reaction mixture, facilitating catalyst recovery and reuse.
Examples:
- Metal Catalysts: Platinum (Pt) or Palladium (Pd) in catalytic converters reduce harmful emissions from car exhausts.
- Zeolites: Microporous, aluminosilicate minerals used in petroleum refining to catalyze hydrocracking.
- Iron in Haber Process: Iron (Fe) is used to catalyze the synthesis of ammonia (NH₃) from nitrogen (N₂) and hydrogen (H₂).
Activation Energy and Reaction Pathway
Activation Energy
Activation energy (Ea) is the minimum amount of energy required for reactants to undergo a chemical reaction. It represents the energy barrier that must be overcome for reactants to transform into products.
- High Activation Energy: Reactions with high activation energies are slower because fewer molecules have the required energy to react.
- Low Activation Energy: Reactions with low activation energies are faster because more molecules have the energy to overcome the energy barrier.
Reaction Pathway
The reaction pathway is the sequence of steps that reactants follow to convert into products. This pathway involves an energy profile that includes the activation energy and transition states.
Energy Diagram
An energy diagram illustrates the energy changes during a chemical reaction. It typically shows the energy of the reactants, the energy of the products, and the activation energy.
Features of an Energy Diagram:
- Reactants: The starting materials of the reaction, typically shown on the left side of the diagram.
- Products: The substances formed as a result of the reaction, typically shown on the right side of the diagram.
- Activation Energy (Ea): The peak of the curve represents the transition state, and the energy difference between the reactants and this peak is the activation energy.
- Transition State: The highest energy point along the reaction pathway, where old bonds are breaking, and new bonds are forming.
- ΔH (Enthalpy Change): The difference in energy between the reactants and products, indicating whether the reaction is exothermic (releases energy) or endothermic (absorbs energy).
Catalysts and Activation Energy
Catalysts provide an alternative reaction pathway with a lower activation energy, thus increasing the reaction rate.
Catalyzed vs. Uncatalyzed Reactions:
- Uncatalyzed Reaction: The energy diagram shows a single pathway with a high activation energy peak.
- Catalyzed Reaction: The energy diagram shows an alternative pathway with a lower activation energy peak.
Energy Diagram Example:
- Without Catalyst:
- High activation energy peak.
- Slower reaction rate.
- With Catalyst:
- Lower activation energy peak.
- Faster reaction rate.
Enzymes as Biological Catalysts
Enzymes are proteins that act as biological catalysts, speeding up biochemical reactions in living organisms with remarkable specificity and efficiency. They play a critical role in various metabolic processes.
Key Characteristics of Enzymes
- Substrate Specificity:
- Enzymes are highly specific, typically catalyzing only one type of reaction or acting on a specific substrate.
- This specificity arises from the enzyme’s active site, a unique region where the substrate binds.
- Active Site:
- The active site is a specially shaped pocket on the enzyme where the substrate fits.
- The active site’s shape and chemical environment are tailored to facilitate the reaction.
- Lock-and-Key Model:
- This model suggests that the enzyme’s active site (the “lock”) is precisely shaped to fit the substrate (the “key”).
- This exact fit ensures high specificity.
- Induced Fit Model:
- This model proposes that the enzyme changes shape slightly to accommodate the substrate.
- The binding of the substrate induces a conformational change in the enzyme, enhancing the interaction and stabilizing the transition state.
Mechanism of Enzyme Action
- Substrate Binding:
- The substrate binds to the enzyme’s active site, forming an enzyme-substrate complex.
- Transition State Stabilization:
- The enzyme stabilizes the transition state, lowering the activation energy required for the reaction.
- Product Formation:
- The reaction occurs, converting the substrate into products.
- Product Release:
- The products are released from the active site, and the enzyme is free to bind to new substrate molecules.
Importance of Enzymes
- Metabolic Regulation: Enzymes regulate metabolic pathways by controlling the rates of biochemical reactions.
- Efficiency: Enzymes can increase reaction rates by factors of millions, making biological processes occur at a pace compatible with life.
- Temperature and pH Sensitivity: Enzymes function optimally within specific temperature and pH ranges, beyond which their activity diminishes.
Catalysis and Reaction Rates
Catalysts play a significant role in altering the rates of chemical reactions by providing an alternative pathway with a lower activation energy. This section explores how catalysis affects reaction rates and the principles behind it.
Rate Laws and Catalysts
Rate laws express the relationship between the rate of a chemical reaction and the concentration of its reactants. Catalysts influence the rate constant (k) in the rate law but do not change the overall stoichiometry of the reaction.
Effect of Catalysts on Reaction Rates
- Lowering Activation Energy:
- Catalysts lower the activation energy (Ea) needed for a reaction to occur.
- With a lower activation energy, more reactant molecules possess the necessary energy to reach the transition state and form products, thereby increasing the reaction rate.
- Alternative Pathway:
- Catalysts provide an alternative reaction pathway with a lower activation energy.
- This alternative pathway often involves the formation of intermediate species that are lower in energy than the transition state of the uncatalyzed reaction.
Mechanisms of Catalyzed Reactions
Catalyzed reactions often proceed through a series of intermediate steps, which can be understood through the following mechanisms:
- Adsorption (Heterogeneous Catalysis):
- Reactants adsorb onto the catalyst’s surface, where the reaction occurs.
- Adsorption weakens reactant bonds, lowering activation energy.
- Intermediate Complex Formation (Homogeneous Catalysis):
- The catalyst forms a temporary intermediate complex with the reactants.
- This complex lowers the activation energy and facilitates the reaction.
Examples of Catalytic Reactions
1. The Haber Process
Reaction: Synthesis of ammonia (NH₃) from nitrogen (N₂) and hydrogen (H₂).
- Equation:
- Catalyst: Iron (Fe).
2. Catalytic Converters
Reaction: Conversion of harmful vehicle exhaust gases into less harmful substances.
- Oxidation of Carbon Monoxide (CO) to Carbon Dioxide (CO₂):
- Reduction of Nitrogen Oxides (NOx) to Nitrogen (N₂):
- Catalysts: Platinum (Pt), palladium (Pd), and rhodium (Rh).