Learning Objectives
For the AP Chemistry exam, you should understand the fundamental principles of galvanic (voltaic) and electrolytic cells. This includes knowing how each type of cell converts energy, the components involved (anode, cathode, electrolyte, salt bridge, and external circuit for galvanic cells; anode, cathode, electrolyte, and power source for electrolytic cells), and the specific redox reactions that occur at each electrode. Be able to differentiate between the spontaneous reactions in galvanic cells and the non-spontaneous reactions in electrolytic cells. Additionally, you should be familiar with the cell notation for galvanic cells, the applications of each cell type, and key concepts such as electromotive force (EMF) and Faraday’s laws of electrolysis.
Introduction
Galvanic (voltaic) cells and electrolytic cells are two types of electrochemical cells that convert chemical energy into electrical energy and vice versa. Galvanic cells generate electricity through spontaneous redox reactions, powering devices like batteries. In contrast, electrolytic cells use an external electrical source to drive non-spontaneous chemical reactions, common in processes like electroplating and the electrolysis of water. Both types of cells involve the movement of electrons through an external circuit and the flow of ions within the electrolyte solution, playing crucial roles in various industrial and technological applications.
Galvanic (Voltaic) Cells
What are Galvanic (Voltaic) Cells?
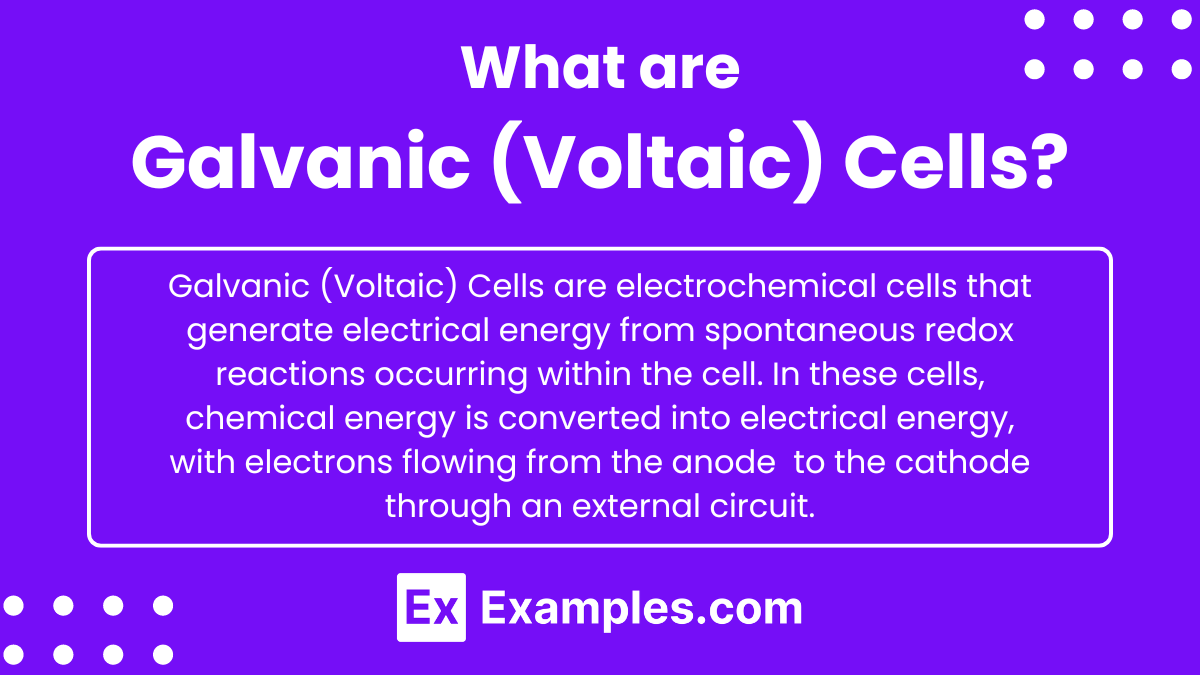
Galvanic (Voltaic) Cells are electrochemical cells that generate electrical energy from spontaneous redox reactions occurring within the cell. In these cells, chemical energy is converted into electrical energy, with electrons flowing from the anode (where oxidation occurs) to the cathode (where reduction occurs) through an external circuit. This process is used in batteries and other devices to provide a continuous source of electrical power.
Diagram of Galvanic Cell
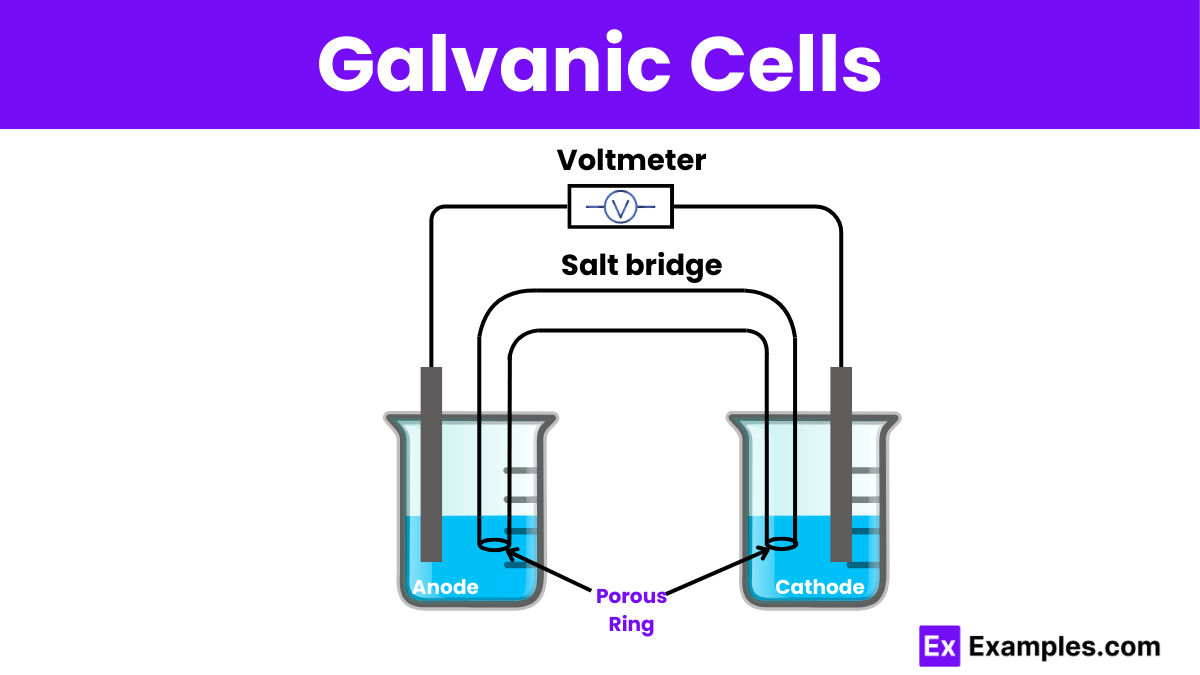
Components of Galvanic Cells
1. Anode
- Function: The anode is the electrode where oxidation occurs. Oxidation is the process of losing electrons. In a galvanic cell, the anode is the site of this loss of electrons, which are then sent through the external circuit.
- Charge: The anode is negatively charged in a galvanic cell. This negative charge is due to the accumulation of electrons released during the oxidation reaction.
- Reaction: At the anode, a chemical species loses electrons. For example, zinc metal oxidizes to form zinc ions and releases electrons:
2. Cathode
- Function: The cathode is the electrode where reduction occurs. Reduction is the process of gaining electrons. Electrons that travel through the external circuit enter the cathode, where they participate in reduction reactions.
- Charge: The cathode is positively charged in a galvanic cell. This positive charge results from the consumption of electrons during the reduction reactions.
- Reaction: At the cathode, a chemical species gains electrons. For example, copper ions in solution are reduced to form copper metal:
3. Salt Bridge
- Function: The salt bridge maintains electrical neutrality within the internal circuit. It connects the two half-cells, allowing the flow of ions while preventing the solutions from mixing directly.
- Composition: Typically, a salt bridge contains a salt solution such as potassium nitrate KNO₃ or sodium sulfate Na₂SO₄. These salts provide ions that can move to balance the charges generated by the oxidation and reduction reactions.
- Importance: Without the salt bridge, the solutions in the half-cells would become electrically unbalanced, as the oxidation reaction produces positive ions and the reduction reaction consumes positive ions. The salt bridge allows negative ions to flow into the anode compartment and positive ions to flow into the cathode compartment, maintaining charge balance.
4. Electrolyte Solutions
- Function: Electrolyte solutions contain the ions that participate in the redox reactions at the electrodes. They provide the medium for the ionic species to move and conduct electricity within the cell.
- Role: These solutions facilitate the transfer of ions to and from the electrodes, which is essential for the redox reactions to occur. The concentration of these ions can affect the cell’s voltage and overall efficiency.
5. External Circuit
- Function: The external circuit connects the anode and cathode, allowing electrons to flow between them. It often includes a load, such as a light bulb or resistor, which harnesses the electrical energy produced by the cell.
- Components: The external circuit is typically a wire or another conductive pathway that provides a route for electron flow.
- Flow of Electrons: In the external circuit, electrons flow from the anode to the cathode. This flow generates an electric current that can be used to perform work.
6. Half-Cells
- Function: Each half-cell consists of an electrode immersed in an electrolyte solution. The two half-cells are where the oxidation and reduction reactions occur.
- Role: Half-cells are essential for separating the oxidation and reduction reactions, allowing the electron flow to be harnessed as electrical energy. Each half-cell contains an electrode and an electrolyte solution that participates in the respective redox reaction.
Working Principle of Galvanic Cells
Galvanic (voltaic) cells convert chemical energy into electrical energy through spontaneous redox reactions.
1. Oxidation at the Anode
- Process: The anode undergoes oxidation, where a chemical species loses electrons.
- Reaction Example: For a zinc anode, the oxidation reaction is:
- Electron Flow: The electrons released during oxidation accumulate at the anode, making it negatively charged.
2. Electron Flow Through the External Circuit
- Flow Direction: Electrons flow from the anode to the cathode through an external circuit.
- Current Generation: This flow of electrons generates an electric current that can be harnessed to do work, such as lighting a bulb or powering a device.
3. Reduction at the Cathode
- Process: The cathode undergoes reduction, where a chemical species gains electrons.
- Reaction Example: For a copper cathode, the reduction reaction is:
- Electron Consumption: The electrons that flow to the cathode are consumed in the reduction reaction, making the cathode positively charged.
4. Ion Flow Through the Salt Bridge
- Purpose: The salt bridge maintains electrical neutrality within the cell by allowing ions to flow between the two half-cells.
- Ion Movement:
- Anions (negative ions) move toward the anode compartment to balance the positive charge generated by the loss of cations.
- Cations (positive ions) move toward the cathode compartment to balance the negative charge generated by the gain of electrons.
5. Maintaining Electrical Neutrality
- Salt Bridge Composition: Typically contains a salt solution like potassium nitrate (KNO(_3)) that dissociates into ions.
- Ion Exchange: The movement of ions through the salt bridge prevents the build-up of charge in either half-cell, which would otherwise stop the flow of electrons and halt the cell’s operation.
The overall reaction in a galvanic cell is the sum of the oxidation reaction at the anode and the reduction reaction at the cathode. For the example reactions given:
Electromotive Force (EMF)
- Definition: The electromotive force (EMF) is the voltage generated by the galvanic cell.
- Measurement: EMF can be measured using a voltmeter connected to the external circuit.
- Dependence: EMF depends on the nature of the materials used for the electrodes and the concentration of the electrolyte solutions.
Examples of Galvanic Cells
1. Daniell Cell
- Components: Zinc anode, copper cathode, zinc sulfate and copper sulfate electrolytes, salt bridge.
- Reactions:
- Anode:
- Cathode:
- Overall Reaction:
- Applications: Educational demonstrations, early battery technology.
2. Leclanché Cell (Dry Cell)
- Components: Zinc anode, carbon cathode with manganese dioxide, ammonium chloride paste, salt bridge.
- Reactions:
- Anode:
- Cathode:
- Overall Reaction:
- Applications: Household batteries, portable devices.
Electrolytic Cells
What are Electrolytic Cells?
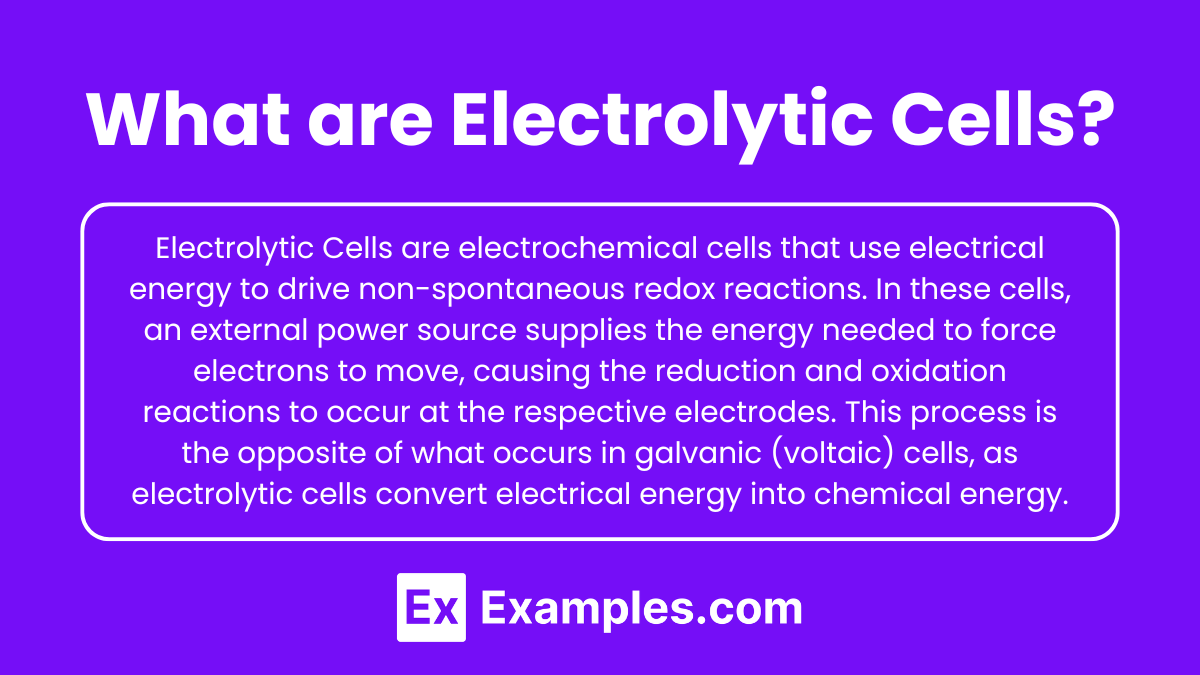
Electrolytic Cells are electrochemical cells that use electrical energy to drive non-spontaneous redox reactions. In these cells, an external power source supplies the energy needed to force electrons to move, causing the reduction and oxidation reactions to occur at the respective electrodes. This process is the opposite of what occurs in galvanic (voltaic) cells, as electrolytic cells convert electrical energy into chemical energy.
Diagram of Electrolytic Cell
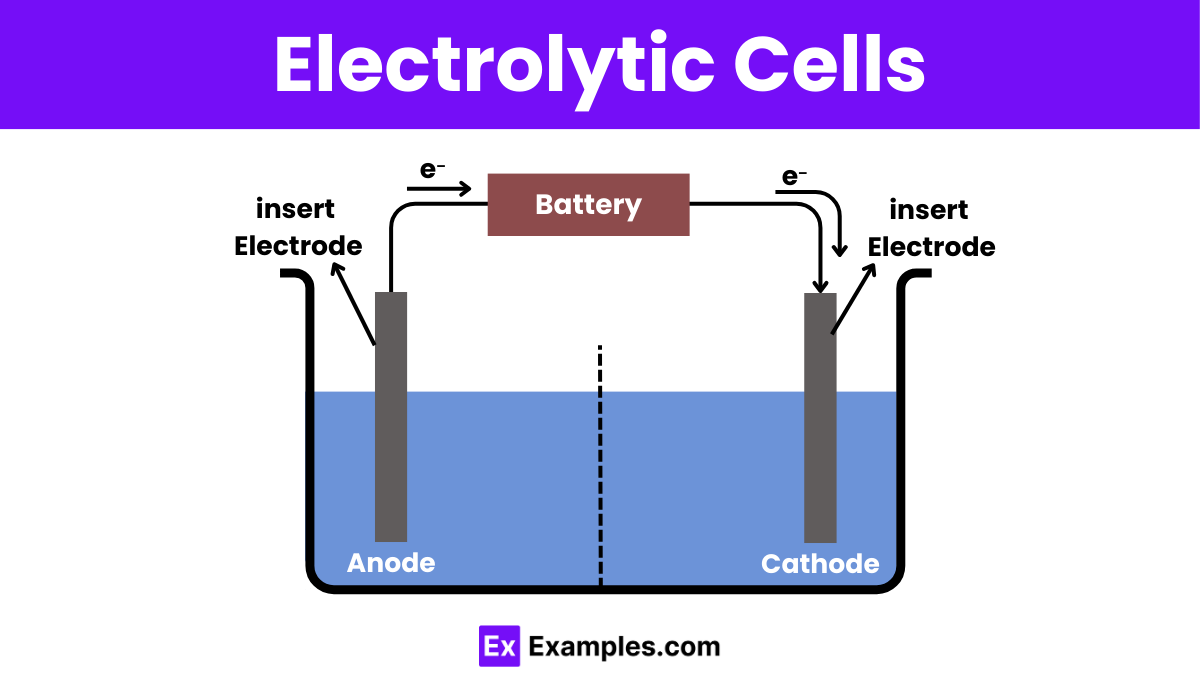
Components of Electrolytic Cells
1. Anode
- Function: The anode is the electrode where oxidation occurs.
- Charge: It is positively charged in an electrolytic cell.
- Reaction: At the anode, a chemical species loses electrons (is oxidized). For example, in the electrolysis of water, oxygen gas is produced at the anode.
2. Cathode
- Function: The cathode is the electrode where reduction occurs.
- Charge: It is negatively charged in an electrolytic cell.
- Reaction: At the cathode, a chemical species gains electrons (is reduced). For example, in the electrolysis of water, hydrogen gas is produced at the cathode.
3. Electrolyte Solution
- Function: The electrolyte solution contains ions that facilitate the redox reactions at the electrodes. It provides a medium for the transfer of ions to and from the electrodes.
- Example: In the electrolysis of water, the electrolyte is often a dilute sulfuric acid solution
or sodium hydroxide
.
4. Power Source
- Function: The power source supplies the electrical energy required to drive the non-spontaneous redox reactions. It forces electrons to move from the anode to the cathode, enabling oxidation and reduction to occur.
- Example: A battery or a direct current (DC) power supply is commonly used as the power source.
5. Electrodes
- Function: Electrodes are conductors through which electrons enter or leave the electrolyte. They serve as sites for oxidation and reduction reactions.
- Materials: Electrodes can be made of various materials such as platinum, graphite, or other inert conductors, depending on the specific application and reactions involved.
6. Reaction Vessel
- Function: The reaction vessel contains the electrolyte solution and the electrodes. It must be made of a material that does not react with the electrolyte or the products of the electrolysis.
- Materials: Common materials for reaction vessels include glass, plastic, or other inert substances.
Working Principle of Electrolytic Cells
Electrolytic cells use electrical energy to drive non-spontaneous redox reactions. Here’s a detailed explanation of how they work:
1. External Power Source
- Function: An external power source, such as a battery or direct current (DC) power supply, provides the necessary electrical energy to drive the redox reactions.
- Voltage Application: The power source applies a voltage across the electrodes, forcing electrons to flow through the external circuit.
2. Oxidation at the Anode
- Process: The anode is the electrode where oxidation occurs. Oxidation involves the loss of electrons.
- Reaction: At the anode, a chemical species loses electrons and is oxidized. For example, during the electrolysis of water:
- Electron Movement: The electrons produced at the anode are pushed into the external circuit by the power source.
3. Reduction at the Cathode
- Process: The cathode is the electrode where reduction occurs. Reduction involves the gain of electrons.
- Reaction: At the cathode, a chemical species gains electrons and is reduced. For example, during the electrolysis of water:
- Electron Movement: Electrons enter the cathode from the external circuit, driven by the power source.
4. Electrolyte Solution
- Function: The electrolyte solution contains ions that facilitate the redox reactions at the electrodes and provides a medium for the transfer of ions between the anode and cathode.
- Ion Movement:
- Cations (positive ions) move toward the cathode to gain electrons and be reduced.
- Anions (negative ions) move toward the anode to lose electrons and be oxidized.
5. Maintaining Charge Balance
- Ion Flow: To maintain electrical neutrality in the cell, ions in the electrolyte move towards the respective electrodes.
- Example: In the electrolysis of water, hydrogen ions ((\text{H}^+)) move towards the cathode, and hydroxide ions
move towards the anode.
6. Reaction
- Combination of Half-Reactions: The overall reaction in an electrolytic cell is the sum of the oxidation and reduction half-reactions.
- Example: For the electrolysis of water:
- Anode Reaction (Oxidation):
- Cathode Reaction (Reduction):
- Reaction:
Examples of Electrolytic Cells
1. Electrolysis of Water
- Components:
- Anode: Inert electrode (e.g., platinum)
- Cathode: Inert electrode (e.g., platinum)
- Electrolyte: Water with acid or base
- Power Source: DC power supply
- Reactions:
- Anode:
- Cathode:
- Overall Reaction:
- Applications: Producing hydrogen and oxygen gases.
2. Electroplating
- Components:
- Anode: Metal to be plated
- Cathode: Object to be plated
- Electrolyte: Solution with metal ions
- Power Source: DC power supply
- Reactions:
- Anode:
- Cathode:
- Overall Reaction:
- Applications: Coating objects with metal.
Differences between Galvanic and Electrolytic Cells
Feature | Galvanic (Voltaic) Cell | Electrolytic Cell |
---|---|---|
Energy Conversion | Chemical to Electrical | Electrical to Chemical |
Reaction Type | Spontaneous | Non-spontaneous |
Anode Charge | Negative (Oxidation) | Positive (Oxidation) |
Cathode Charge | Positive (Reduction) | Negative (Reduction) |
External Power Source | Not required | Required |
Electron Flow | From Anode to Cathode through external circuit | From Power Source to Cathode |
Ion Flow | Through Salt Bridge to maintain charge balance | Through Electrolyte to maintain charge balance |
Applications | Batteries, fuel cells | Electroplating, electrolysis of compounds, metal extraction |
Example | Daniell Cell | Electrolysis of Water, Electroplating, Hall-Héroult Process |
- Galvanic Cells generate electrical energy from spontaneous redox reactions, do not require an external power source, and have a negative anode and positive cathode.
- Electrolytic Cells use electrical energy to drive non-spontaneous redox reactions, require an external power source, and have a positive anode and negative cathode.