Learning Objectives
For the AP Chemistry exam, you should aim to understand and differentiate between the types of intramolecular forces, including covalent, ionic, and metallic bonds. Analyze how bond strength, bond length, and bond energy relate to potential energy within a molecule. Apply concepts of electronegativity, atomic size, and bond order to predict and explain bond properties and behaviors. Evaluate the role of intramolecular forces in chemical reactions, particularly how bond formation and breaking affect the system’s potential energy. Utilize Coulomb’s law to describe and calculate the potential energy of ionic interactions. Explain the electron sea model in metallic bonding and its implications for the physical properties of metals. Connect intramolecular forces to reaction energetics, understanding exothermic and endothermic processes in terms of bond energies. Mastering these objectives will provide a comprehensive understanding of how intramolecular forces govern the stability, reactivity, and energy changes in chemical systems.
Introduction
Intramolecular forces are the forces that hold atoms together within a molecule, including covalent, ionic, and metallic bonds. These forces are crucial because they determine the structure, stability, and chemical properties of molecules. Potential energy within a molecule is directly related to these bonds—the stronger the bond, the lower the potential energy and the more stable the molecule. Understanding how these forces work and how they influence potential energy helps explain why molecules behave the way they do in chemical reactions. This knowledge is essential for mastering concepts in AP Chemistry.
Intramolecular Force
What are Intramolecular Forces?
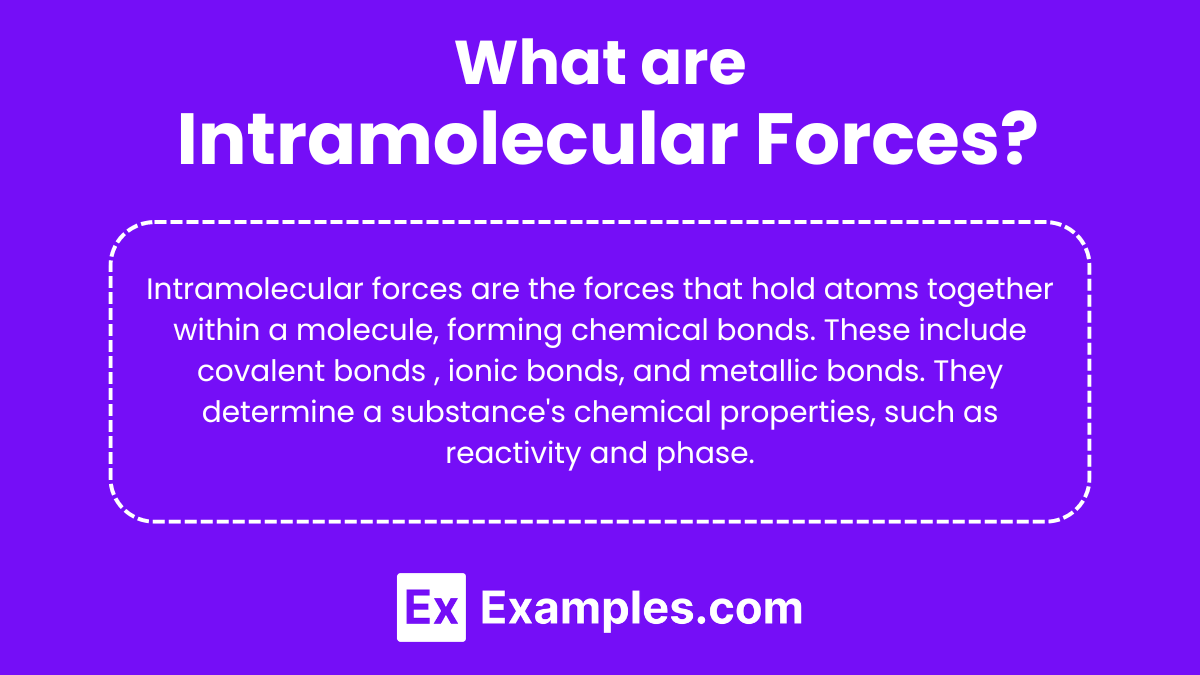
Intramolecular forces are the forces that hold atoms together within a molecule, forming the chemical bonds that result in molecular structures. These forces include covalent bonds, where atoms share pairs of electrons; ionic bonds, created by the electrostatic attraction between oppositely charged ions; and metallic bonds, which involve a “sea of electrons” shared among many metal nuclei. Intramolecular forces are essential for determining a substance’s chemical properties, such as reactivity, polarity, phase (solid, liquid, gas), and color.
Types of Intramolecular Forces
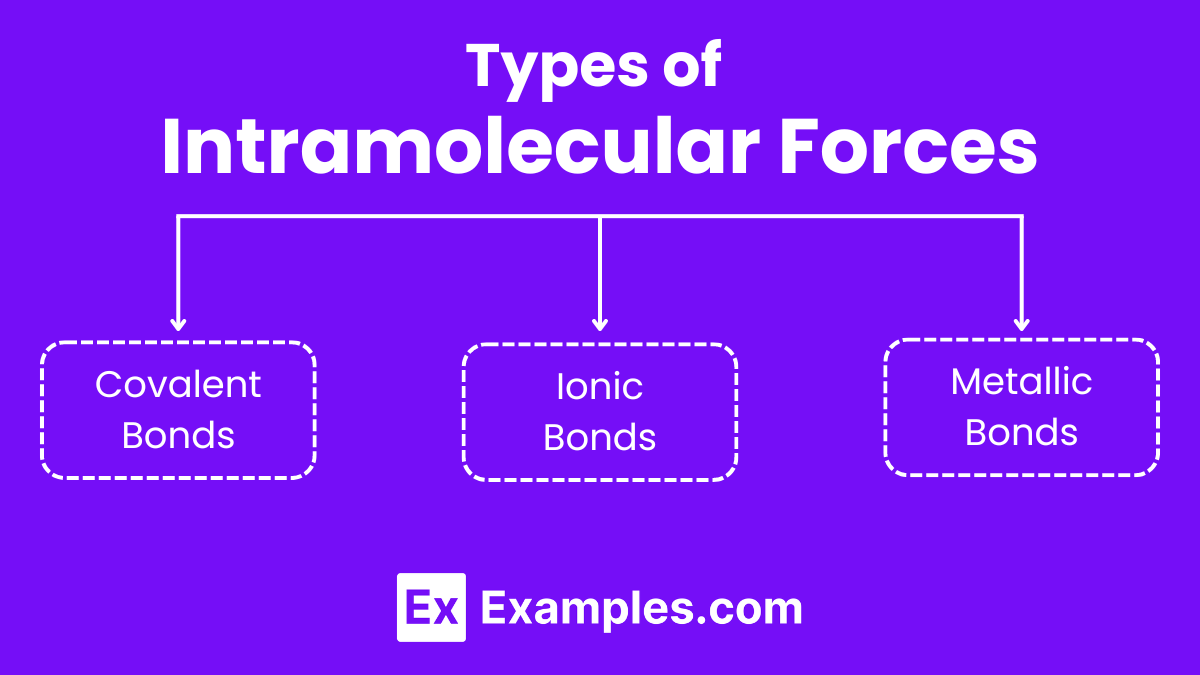
Covalent Bonds
Covalent bonds form when two atoms share one or more pairs of electrons to achieve stable electron configurations, typically resulting in a molecule with lower potential energy. This type of bonding usually occurs between nonmetal atoms with similar electronegativities.
- Single Covalent Bond: Involves the sharing of one pair of electrons between two atoms.
- Example: Hydrogen Molecule (H₂): Each hydrogen atom contributes one electron to form a single bond, resulting in a stable H₂ molecule.
- Double Covalent Bond: Involves the sharing of two pairs of electrons between two atoms.
- Example: Oxygen Molecule (O₂): Each oxygen atom shares two electrons with the other, forming a double bond that creates a stable O₂ molecule.
- Triple Covalent Bond: Involves the sharing of three pairs of electrons between two atoms.
- Example: Nitrogen Molecule (N₂): Each nitrogen atom shares three electrons with the other, forming a triple bond, making N₂ one of the strongest and most stable diatomic molecules.
Ionic Bonds
Ionic bonds form when electrons are transferred from one atom to another, creating ions with opposite charges that attract each other. This type of bond generally occurs between metal and nonmetal atoms, where one atom (typically a metal) loses electrons to become a positively charged ion (cation), and the other atom (typically a nonmetal) gains those electrons to become a negatively charged ion (anion).
- Example: Sodium Chloride (NaCl): Sodium (Na) donates one electron to chlorine (Cl), resulting in the formation of a Na⁺ ion and a Cl⁻ ion. The electrostatic attraction between these oppositely charged ions forms the ionic bond, creating the compound NaCl.
- Example: Magnesium Oxide (MgO): Magnesium (Mg) donates two electrons to oxygen (O), resulting in a Mg²⁺ ion and an O²⁻ ion. The strong electrostatic attraction between these ions forms a stable ionic compound, MgO.
Metallic Bonds
Metallic bonds occur between metal atoms, where the outer electrons are not bound to any specific atom and can move freely throughout the entire structure. This “sea of electrons” provides metals with their characteristic properties such as electrical conductivity, malleability, and ductility. The positively charged metal ions are held together by the negatively charged delocalized electrons.
- Example: Copper (Cu): Copper atoms are arranged in a lattice structure, with a sea of delocalized electrons flowing freely around them. This allows copper to conduct electricity and heat efficiently.
- Example: Iron (Fe): In iron, the metal atoms are surrounded by a sea of delocalized electrons that hold the atoms together in a metallic lattice. This gives iron its strength, malleability, and ability to conduct heat and electricity.
Intramolecular Forces and Chemical Reactions
Intramolecular forces play a crucial role in determining how chemical reactions occur and the energy changes associated with them. These forces, which include covalent, ionic, and metallic bonds, influence the stability, reactivity, and energy dynamics of molecules and compounds during chemical reactions.
Role of Intramolecular Forces in Chemical Reactions
- Bond Formation and Bond Breaking
- Bond Formation: When new bonds are formed during a chemical reaction, energy is released. This energy release occurs because the system transitions to a more stable state with lower potential energy. The strength of the newly formed bonds determines the amount of energy released. Stronger bonds release more energy.
- Bond Breaking: To initiate a chemical reaction, existing bonds must be broken. This process requires an input of energy, known as bond dissociation energy. The amount of energy required to break a bond depends on its strength; stronger bonds require more energy to break.
- Energy Changes (Enthalpy)
- Exothermic Reactions: These reactions release energy, primarily because the energy released during bond formation exceeds the energy required to break the initial bonds. The products are typically more stable (lower potential energy) than the reactants.
- Example: Combustion of methane (CH₄ + 2O₂ → CO₂ + 2H₂O) releases a large amount of energy as heat.
- Endothermic Reactions: These reactions absorb energy, as the energy required to break the initial bonds is greater than the energy released during the formation of new bonds. The products are less stable (higher potential energy) than the reactants.
- Example: Decomposition of calcium carbonate (CaCO₃ → CaO + CO₂) requires continuous energy input.
- Exothermic Reactions: These reactions release energy, primarily because the energy released during bond formation exceeds the energy required to break the initial bonds. The products are typically more stable (lower potential energy) than the reactants.
- Reaction Mechanisms
- Transition States and Activation Energy: The energy required to initiate a reaction (activation energy) is influenced by the strength of intramolecular forces. Reactions involving the breaking of strong bonds have higher activation energies. Transition states, which represent high-energy configurations during a reaction, also depend on the nature of the bonds being formed and broken.
- Catalysts: Catalysts can lower the activation energy by providing an alternative reaction pathway with different bond interactions. This facilitates the breaking and forming of bonds more efficiently.
- Chemical Equilibrium
- Dynamic Nature: In a reversible reaction, the formation and breaking of bonds occur simultaneously. The relative strengths of these bonds determine the position of equilibrium. Stronger bonds in the products will shift the equilibrium towards the product side, while stronger bonds in the reactants will favor the reactants.
- Le Chatelier’s Principle: Changes in conditions such as temperature, pressure, and concentration can affect the equilibrium position by altering the balance of bond energies.
- Molecular Stability
- Bond Strength and Stability: Molecules with stronger intramolecular forces (e.g., triple bonds in N₂) are more stable and less reactive. Conversely, molecules with weaker bonds (e.g., single bonds in alkanes) are generally more reactive.
- Resonance and Delocalization: In some molecules, delocalized electrons (as in benzene) provide additional stability, influencing the reactivity and the course of chemical reactions.
Examples of Intramolecular Forces
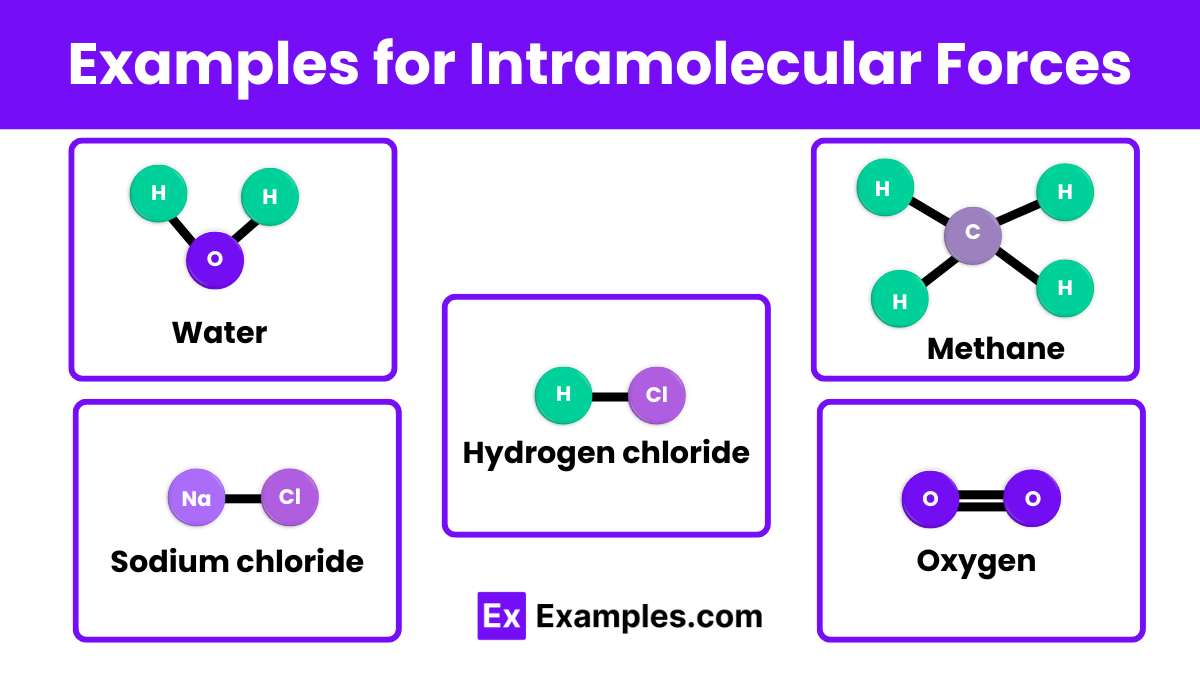
- Hydrogen Bonding: Water (H₂O) molecules attract each other via hydrogen bonds, leading to high boiling points.
- Dipole-Dipole Interactions: Hydrogen chloride (HCl) molecules attract each other through dipole-dipole forces.
- London Dispersion Forces: Methane (CH₄) molecules experience temporary dipoles, causing weak attractions.
- Ion-Dipole Interactions: Sodium chloride (NaCl) dissolves in water with Na⁺ and Cl⁻ ions interacting with water molecules.
- Dipole-Induced Dipole Interactions: Oxygen (O₂) molecules dissolve in water due to induced dipoles from water molecules.
Potential Energy
What is Potential Energy?
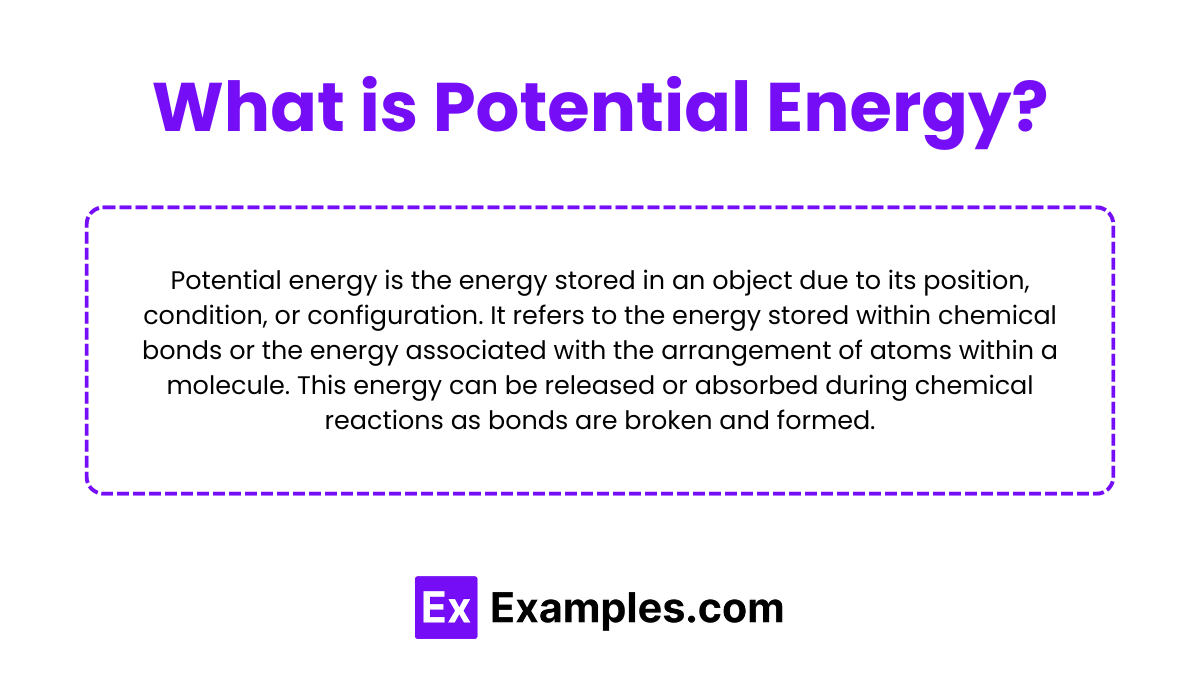
Potential energy is the energy stored in an object due to its position, condition, or configuration. This type of energy has the potential to do work as a result of an object’s position in a force field (such as gravitational, electric, or magnetic) or due to its configuration in a system of objects. Common forms of potential energy include gravitational potential energy, elastic potential energy, and chemical potential energy.
Potential Energy in Intramolecular Forces
Potential energy in intramolecular forces refers to the energy stored within the bonds that hold atoms together in a molecule. This energy is a result of the attractive and repulsive forces between atoms.
Key Points:
- Covalent Bonds:
- Bond Length: The optimal distance between two bonded atoms where the potential energy is at its minimum. If atoms are too close, repulsive forces increase potential energy; if too far, attractive forces weaken, raising potential energy.
- Bond Energy: The energy required to break a bond. Stronger bonds (like double or triple bonds) have higher bond energies and thus lower potential energy when formed.
- Ionic Bonds:
- Lattice Energy: The energy released when positive and negative ions form an ionic compound. Higher lattice energy indicates stronger bonds and lower potential energy within the crystal lattice.
- Coulomb’s Law: Describes the potential energy of the electrostatic attraction between oppositely charged ions. The potential energy decreases as the charges of the ions increase or the distance between them decreases.
- Metallic Bonds:
- Electron Sea Model: Describes how electrons are delocalized among metal atoms, resulting in a strong bond with lower potential energy. The movement of these electrons provides metals with their characteristic properties, such as conductivity and malleability.
Potential Energy in Chemical Bonds
Potential energy in chemical bonds refers to the energy stored within the bonds that hold atoms together in a molecule. This energy is due to the electrostatic forces of attraction between the positively charged nuclei and the negatively charged electrons. When bonds are formed, energy is released, lowering the system’s potential energy. Conversely, when bonds are broken, energy is absorbed, increasing the system’s potential energy.
Factors Affecting Bond Strength and Potential Energy
Bond Length:
- Definition: The distance between the nuclei of two bonded atoms.
- Impact: Shorter bond lengths generally result in stronger bonds and lower potential energy because the attractive forces between the nuclei and electrons are stronger.
Bond Order:
- Definition: The number of shared electron pairs between two atoms.
- Impact: Higher bond order (e.g., double or triple bonds) results in stronger bonds and lower potential energy. For example, a triple bond is stronger and has lower potential energy than a double bond.
Electronegativity:
- Definition: The ability of an atom to attract shared electrons.
- Impact: A greater difference in electronegativity between two bonded atoms typically leads to stronger bonds (ionic character) and lower potential energy.
Atomic Size:
- Definition: The size of the bonded atoms.
- Impact: Smaller atoms with less distance between their nuclei and bonding electrons form stronger bonds with lower potential energy compared to larger atoms.
Examples of Potential Energy
- Water (H₂O): The potential energy is stored in the O-H bonds. Breaking these bonds requires energy, while forming them releases energy.
- Sodium Chloride (NaCl): The potential energy is stored in the electrostatic attraction between Na⁺ and Cl⁻ ions. This potential energy is released as lattice energy when the solid ionic compound forms from gaseous ions.
- Copper (Cu): The potential energy is stored in the attraction between positively charged copper ions and the sea of delocalized electrons, giving copper its electrical conductivity.
- Combustion of Methane (CH₄ + 2O₂ → CO₂ + 2H₂O): The potential energy stored in the C-H and O=O bonds is converted to kinetic energy (heat and light) as new bonds (C=O and O-H) form with lower potential energy.
- Adenosine Triphosphate (ATP): The potential energy is stored in the high-energy phosphate bonds. When ATP is hydrolyzed to ADP (adenosine diphosphate), this energy is released to power cellular processes.