Learning Objectives
In this topic, you should be able to define and describe reaction mechanisms, understanding their significance in chemistry. Learn to identify and explain the components of reaction mechanisms, including elementary steps, intermediates, and transition states. Grasp how to write and apply rate laws, identify the rate-determining step, and assess its influence on the overall reaction rate. You should be able to propose and evaluate reaction mechanisms using experimental data, understand and apply collision and transition state theories, and explain the role of catalysts in reactions. Finally, practice solving problems and analyzing real-world examples to solidify your understanding and prepare for the AP Chemistry exam.
Introduction
Reaction mechanisms detail the step-by-step processes of chemical reactions, showing how atoms and molecules interact and transform. This understanding is crucial for predicting reaction outcomes, designing new processes, and advancing fields like organic chemistry and biochemistry.
What is a Reaction Mechanism?
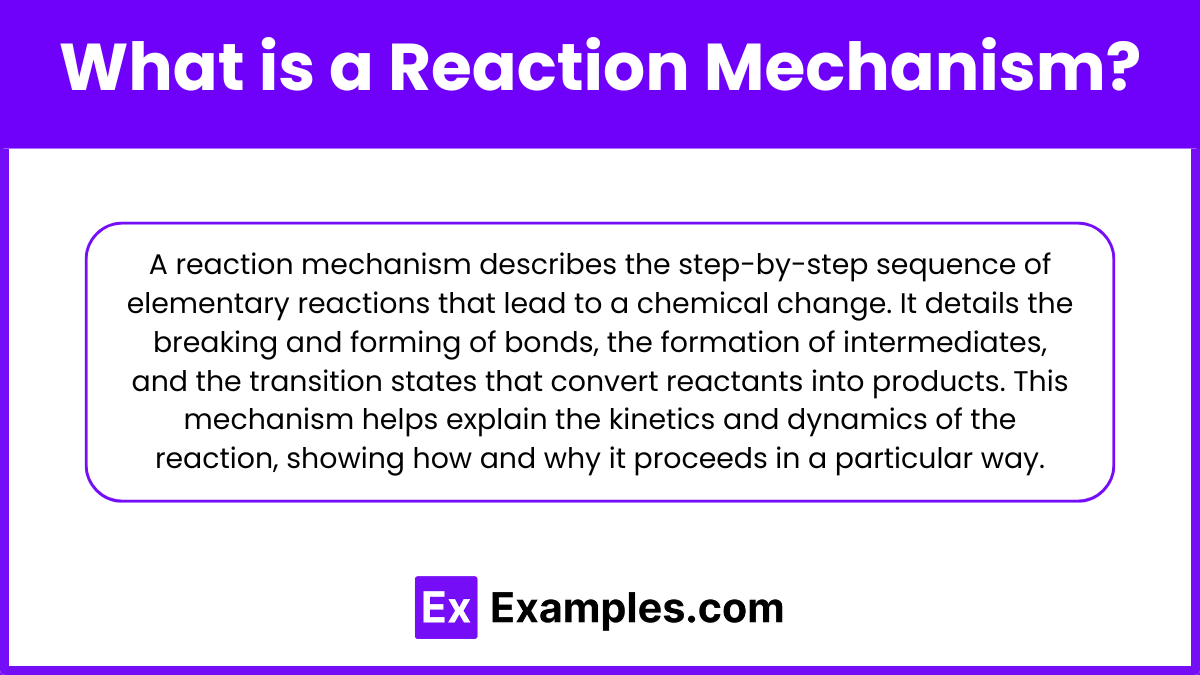
A reaction mechanism is a detailed, step-by-step description of the sequence of elementary reactions by which a chemical change occurs. It outlines the specific molecular events, including the breaking and forming of bonds, the formation of intermediates, and the transition states, that transform reactants into products. This mechanism provides insight into the kinetics and dynamics of a chemical reaction, explaining how and why the reaction proceeds as it does.
Elements of Reaction Mechanisms
1. Elementary Steps
Elementary steps are the individual stages in a reaction mechanism, each representing a single molecular event. They can be classified based on the number of reactant molecules involved:
- Unimolecular: Involves one reactant molecule (e.g., A→BA
BA→B).
- Bimolecular: Involves two reactant molecules (e.g., A+B→CA + B
CA+B→C).
- Termolecular: Involves three reactant molecules (e.g., A+B+C→DA + B + C
DA+B+C→D).
2. Reaction Intermediates
Intermediates are species that are formed in one elementary step and consumed in a subsequent step. They are not present in the overall balanced equation for the reaction but play a crucial role in the mechanism.
3. Transition States
The transition state is a high-energy, unstable arrangement of atoms that occurs during the transformation from reactants to products. It represents the peak of the energy barrier that must be overcome for the reaction to proceed.
4. Rate-Determining Step (RDS)
The rate-determining step is the slowest elementary step in the reaction mechanism, which controls the overall reaction rate. The rate law of the overall reaction is often determined by this step.
5. Molecularity
Molecularity refers to the number of reactant molecules involved in an elementary step:
- Unimolecular: Involves one reactant molecule.
- Bimolecular: Involves two reactant molecules.
- Termolecular: Involves three reactant molecules (rare due to the low probability of three molecules colliding simultaneously).
6. Activation Energy (Ea)
Activation energy is the minimum energy required for the reactants to reach the transition state and proceed to products. It determines the rate at which the reaction occurs.
7. Catalysts
Catalysts are substances that increase the reaction rate without being consumed in the reaction. They provide an alternative pathway with a lower activation energy.
Mechanism Types
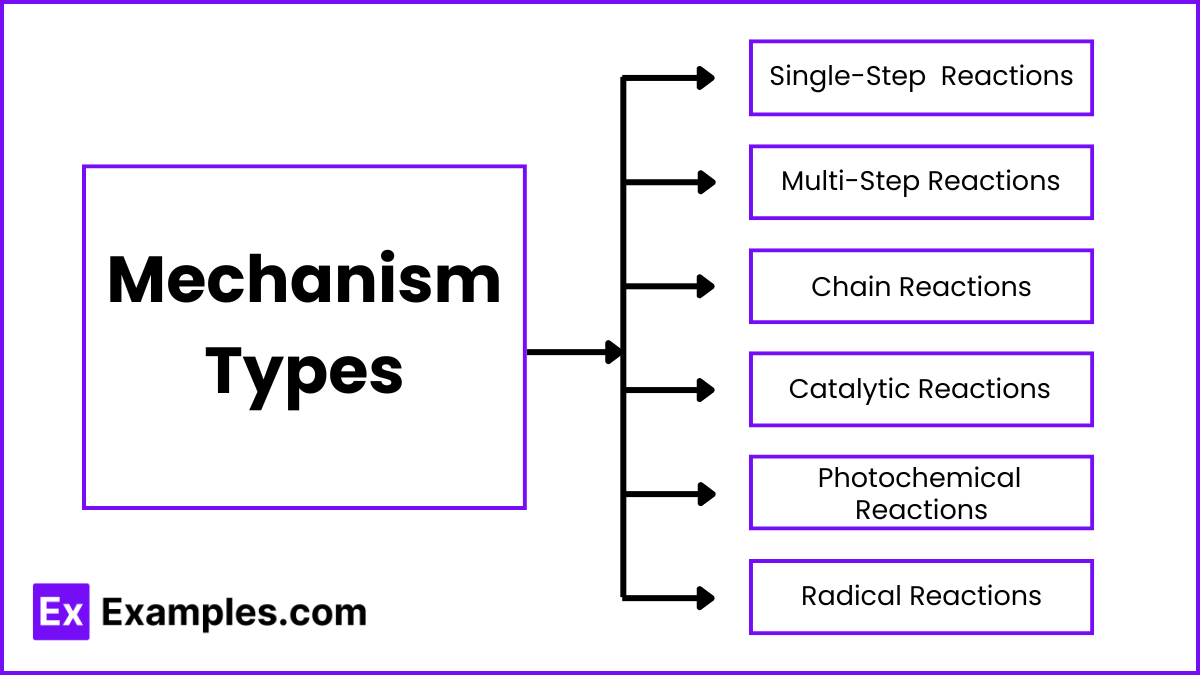
1. Single-Step (Elementary) Reactions
- Definition: These reactions occur in a single elementary step without intermediates.
- Characteristics:
- Involves direct conversion of reactants to products.
- Can be unimolecular, bimolecular, or termolecular.
- Rate law can be directly derived from the stoichiometry of the reaction.
- Example:
- Decomposition of ozone: O₃→ O₂ + O
2. Multi-Step (Complex) Reactions
- Definition: These reactions involve multiple elementary steps, each with its own transition state and intermediate species.
- Characteristics:
- Composed of a sequence of elementary reactions.
- Overall rate is often determined by the slowest step, known as the rate-determining step (RDS).
- Intermediates are formed and consumed during the reaction.
- Example:
- Formation of nitric oxide:
- 2NO₂ → 2NO + O₂ (slow).
- NO + O₃ → NO₂ + O₂ (fast).
- Formation of nitric oxide:
3. Chain Reactions
- Definition: These involve a sequence of reactions where a reactive intermediate generated in one step initiates further reactions.
- Characteristics:
- Comprises initiation, propagation, and termination steps.
- Often seen in polymerizations and combustion reactions.
- Example:
- Chlorination of methane:
- Initiation: Cl₂ → 2Cl⋅.
- Propagation:
- Cl⋅ + CH₄ → HCl + CH₃.
- CH₃⋅ + Cl₂ → CH₃Cl + Cl⋅
- Termination: Cl⋅ + Cl⋅ → Cl₂.
- Chlorination of methane:
4. Catalytic Reactions
- Definition: Reactions that are accelerated by the presence of a catalyst, which provides an alternative pathway with a lower activation energy.
- Characteristics:
- Catalyst is not consumed in the reaction.
- Can involve homogeneous or heterogeneous catalysis.
- Example:
- Hydrogenation of ethene:
- H₂ + C₂H₄ → C₂H₆ in the presence of a platinum catalyst.
- Hydrogenation of ethene:
5. Photochemical Reactions
- Definition: Reactions initiated by the absorption of light (photons).
- Characteristics:
- Involves excited electronic states.
- Common in processes like photosynthesis and vision.
- Example:
- Decomposition of hydrogen peroxide:
- 2H₂O₂ → 2H₂O +O₂ under UV light.
6. Radical Reactions
- Definition: Reactions that involve free radicals, which are highly reactive species with unpaired electrons.
- Characteristics:
- Often involve initiation, propagation, and termination steps.
- Common in polymerization and combustion processes.
- Example:
- Formation of polyethylene:
- Initiation: R⋅ → R⋅.
- Propagation: R⋅ + C₂H₄ → R−C₂H₄⋅.
- Termination: R⋅+ R⋅ → R−R
- Formation of polyethylene:
Rate Laws and Mechanisms
1. Rate Law
- Definition: A rate law is an equation that relates the rate of a chemical reaction to the concentration of its reactants.
- General Form: Rate=k[A]ᵐ[B]ⁿ where k is the rate constant, [A] and [B][\text{B}][B] are the concentrations of the reactants, and mmm and nnn are the reaction orders with respect to each reactant.
2. Determining Rate Laws
- Experimental Determination: Rate laws must be determined experimentally, as they cannot be inferred directly from the stoichiometric coefficients of the overall balanced equation.
- Method: Common methods include the initial rates method, where the reaction rate is measured at the beginning of the reaction for different initial concentrations of reactants.
3. Reaction Order
- Definition: The sum of the exponents mmm and nnn in the rate law gives the overall reaction order.
- Types:
- Zero-Order: The rate is independent of the concentration of reactants.
- Example: Rate = k
- First-Order: The rate is directly proportional to the concentration of one reactant.
- Example: Rate = k[A]
- Second-Order: The rate is proportional to the product of the concentrations of two reactants or the square of the concentration of one reactant.
- Example: Rate = k[A]² or Rate = k[A][B]
- Zero-Order: The rate is independent of the concentration of reactants.
4. Mechanisms and Rate Laws
- Elementary Steps: For an elementary step, the rate law can be written directly from the molecularity (number of molecules involved in the step).
- Unimolecular Step: A → Products Rate = k[A]
- Bimolecular Step: A + B → Products Rate = k[A][B]
- Rate-Determining Step (RDS): The slowest step in a multi-step reaction mechanism, which dictates the overall rate law.
- Example: For a mechanism involving steps:
- A + B → C (slow)C → D (fast)
- The rate law for the overall reaction is determined by the slow step: Rate = k[A] [B].
- Example: For a mechanism involving steps:
5. Steady-State Approximation
- Definition: Assumes that the concentration of intermediates remains constant throughout the reaction.
- Application: Used to simplify the rate law for complex mechanisms.
- Example: For the mechanism:
- A → I (fast equilibrium)I → B (slow)
- The rate law is determined by setting the rate of formation of the intermediate I\text{I}I equal to its rate of consumption.
- Example: For the mechanism:
Step-by-Step Approach to Analyzing Mechanisms
- Write the Overall Reaction: Start with the balanced chemical equation for the overall reaction.
- Propose a Mechanism: Break down the overall reaction into a series of plausible elementary steps that describe the pathway from reactants to products.
- Identify the Rate-Determining Step (RDS): Determine which step is the slowest, as it will control the overall reaction rate.
- Write the Rate Law Based on the RDS: Use the reactants of the rate-determining step to write the rate law expression.
- Check Consistency with Experimental Data: Compare the derived rate law with the experimentally determined rate law to ensure they match.
- Use the Steady-State Approximation if Necessary: Assume the concentration of intermediates remains constant to simplify the rate law for complex mechanisms.
- Verify Molecularity: Ensure the molecularity (number of molecules involved) in each elementary step matches the stoichiometry in the rate law.
- Confirm Mechanism Completeness: Verify that all intermediates are formed and consumed within the steps and that all steps add up to the overall balanced equation.
Examples
1. The Decomposition of Hydrogen Peroxide (H₂O₂)
Overall Reaction:
2H₂O₂ → 2H₂O + O₂
Proposed Mechanism:
- H₂O₂ → HO₂⋅ + H⋅ (slow)
- HO₂⋅ + H₂O₂ → H₂O+ H⋅ + O₂ (fast)
Rate-Determining Step:
The first step is the slow step, which controls the overall rate of the reaction.
Rate Law:
Rate=k[H₂O₂]
2. The Formation of Nitrogen Dioxide (NO₂) from Nitric Oxide (NO) and Oxygen (O₂)
Overall Reaction:
2NO + O₂ → 2NO₂
Proposed Mechanism:
- NO + O₂ → NO₃ (fast equilibrium)
- NO₃ + NO → 2NO₂ (slow)
Rate-Determining Step:
The second step is the slow step, which controls the overall rate of the reaction.
Rate Law:
Rate = k[NO]₂[O₂]