Photoelectric Effect
Learning Objectives
By studying the photoelectric effect, you should be able to define and describe the phenomenon, understand and explain Einstein’s theory involving photons and their energy, calculate photon energy using E=hνE = h \nuE=hν, explain the significance of the work function and threshold frequency, calculate the kinetic energy of emitted electrons using KE=hν−ϕKE = h \nu – \phiKE=hν−ϕ, describe the experimental setup used to observe the effect, analyze factors affecting the effect such as light frequency and intensity and material properties, and identify and explain real-world applications like photocells, light meters, and automatic doors. Understanding these concepts will help you excel in your AP Chemistry exam and grasp fundamental quantum mechanics principles.
Introduction
The photoelectric effect is a fundamental phenomenon in which electrons are emitted from the surface of a material, typically a metal, when it is exposed to light of a certain frequency. This effect, explained by Albert Einstein in 1905, demonstrated that light behaves as both a wave and a particle, leading to significant advancements in quantum mechanics. Understanding the photoelectric effect involves exploring the relationship between light frequency, energy of photons, and the material’s work function, providing key insights into the nature of light and electronic behavior.
What is the Photoelectric Effect?
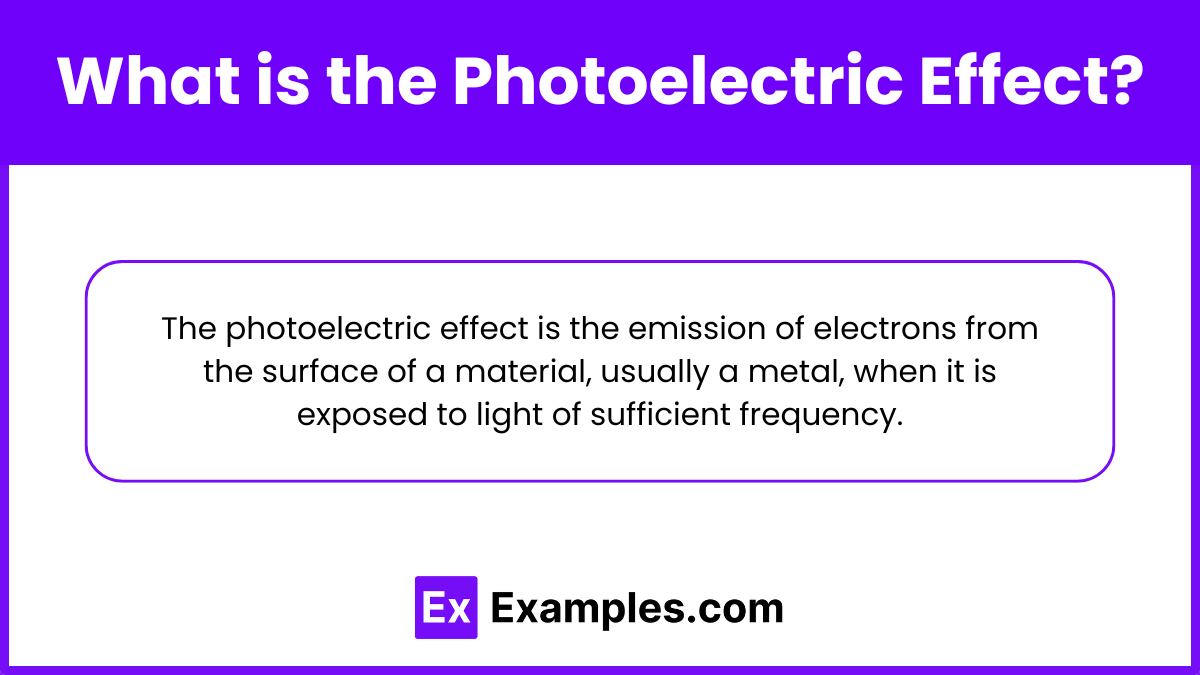
The photoelectric effect is the phenomenon where electrons are emitted from the surface of a material, typically a metal, when it absorbs electromagnetic radiation, such as light, of sufficient frequency. This effect occurs when the energy of the incoming photons exceeds the material’s work function, causing the electrons to be ejected from the surface. The photoelectric effect is a key concept in quantum mechanics, demonstrating the particle nature of light and leading to the development of modern physics theories.
Historical Background
The photoelectric effect has a rich historical background that significantly impacted modern physics. First observed by Heinrich Hertz in 1887, Hertz noticed that ultraviolet light could induce sparks between metal electrodes. Wilhelm Hallwachs built on this discovery in 1888 by demonstrating that ultraviolet light caused a negatively charged zinc plate to lose its charge, suggesting electron emission. Philipp Lenard’s experiments in 1899 further showed that the energy of emitted electrons depended on light frequency, not intensity. Albert Einstein provided the theoretical explanation in 1905, proposing that light consists of photons, each with energy proportional to its frequency. Einstein’s work, which earned him the Nobel Prize in 1921, introduced the concept of wave-particle duality and laid the foundation for quantum mechanics, profoundly shaping our understanding of light and matter interactions.
Einstein’s Explanation
Einstein proposed that light consists of particles called photons. Each photon has energy given by:
\text{E} = \text{h} \nu
Where:
- E is the energy of the photon.
- h is Planck’s constant (6.626×10⁻³⁴ Js).
- ν is the frequency of the light.
Key Equations and Concepts
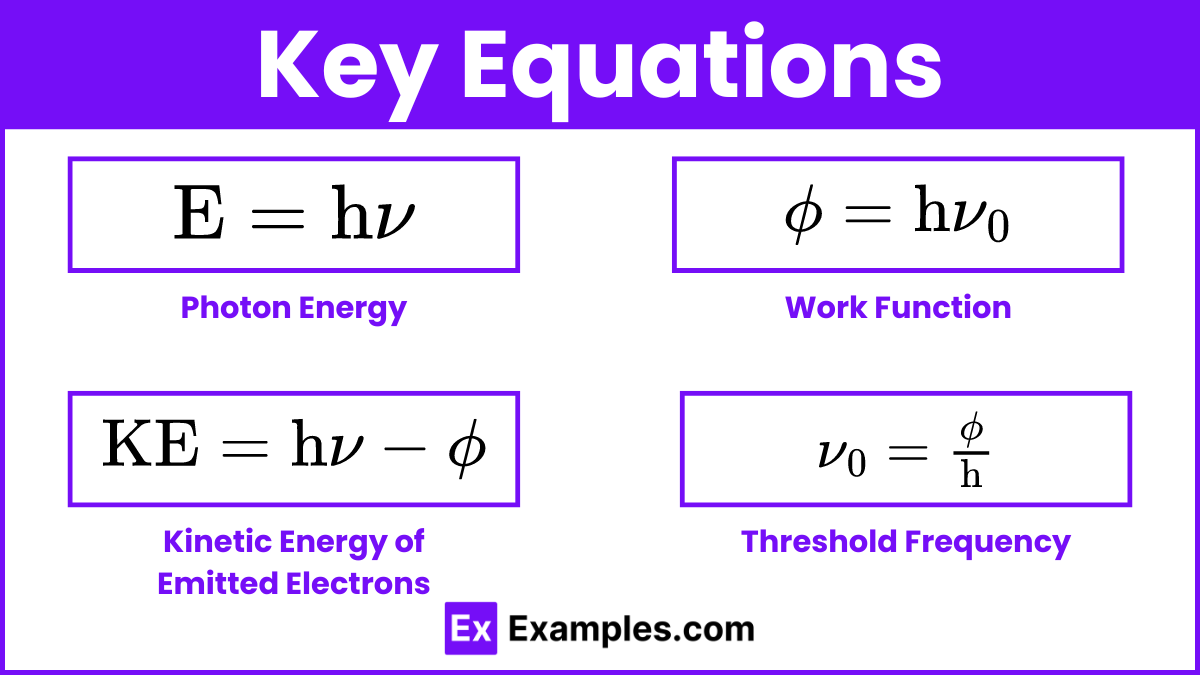
1. Photon Energy
The energy of a photon is directly proportional to its frequency:
\text{E} = \text{h} \nu
Where:
- E is the energy of the photon.
- h is Planck’s constant (6.626×10⁻³⁴ Js).
- ν is the frequency of the light.
2. Work Function
The work function (ϕ) is the minimum energy required to remove an electron from the surface of a material:
\phi = \text{h} \nu_0
Where:
- ϕ is the work function.
- ν₀ is the threshold frequency.
3. Kinetic Energy of Emitted Electrons
If the energy of the incoming photon exceeds the work function, the excess energy is converted into the kinetic energy of the emitted electron:
\text{KE} = \text{h} \nu – \phi
Where:
- KE is the kinetic energy of the emitted electron.
- νis the frequency of the incident light.
- h is Planck’s constant.
- ϕ is the work function.
4. Threshold Frequency
The threshold frequency (ν₀) is the minimum frequency of light required to emit electrons from a material:
\nu_0 = \frac{\phi}{\text{h}}
5. Photoelectric Current
The photoelectric current is the flow of emitted photoelectrons. The number of photoelectrons is directly proportional to the intensity of the incident light, provided the light frequency is above the threshold frequency.
Factors Affecting the Photoelectric Effect
1. Frequency of Light
- Below Threshold Frequency: If the frequency of the incident light (ν) is below the threshold frequency (ν₀), no photoelectrons are emitted, regardless of the light’s intensity.
- Above Threshold Frequency: When the frequency of the light is above the threshold frequency, photoelectrons are emitted. The kinetic energy of the emitted electrons increases with the frequency of the incident light.
2. Intensity of Light
- Below Threshold Frequency: No matter how intense the light is, if its frequency is below the threshold frequency, no electrons are emitted.
- Above Threshold Frequency: If the light frequency is above the threshold frequency, increasing the intensity of the light increases the number of emitted photoelectrons but does not affect their kinetic energy.
3. Work Function of the Material
- Material Dependence: Different materials have different work functions (ϕ). Materials with lower work functions require lower photon energies (and thus lower frequencies) to emit electrons.
- Threshold Frequency: The threshold frequency (ν₀) is specific to each material and is directly related to its work function.
4. Wavelength of Light
- Inverse Relationship: Since frequency (ν) and wavelength (λ) are inversely related (ν = \frac{c}{\lambda}, where c is the speed of light), shorter wavelengths (higher frequencies) are more effective in causing the photoelectric effect. Light with a wavelength longer than the threshold wavelength (\lambda_0 = \frac{c}{\nu_0}) will not cause electron emission.
5. Surface Condition of the Material
- Surface Cleanliness: The presence of oxides or contaminants on the surface can affect the emission of electrons. A clean, polished surface generally yields better results.
- Surface Structure: The atomic arrangement and surface morphology can also influence the efficiency of electron emission.
Applications of the Photoelectric Effect
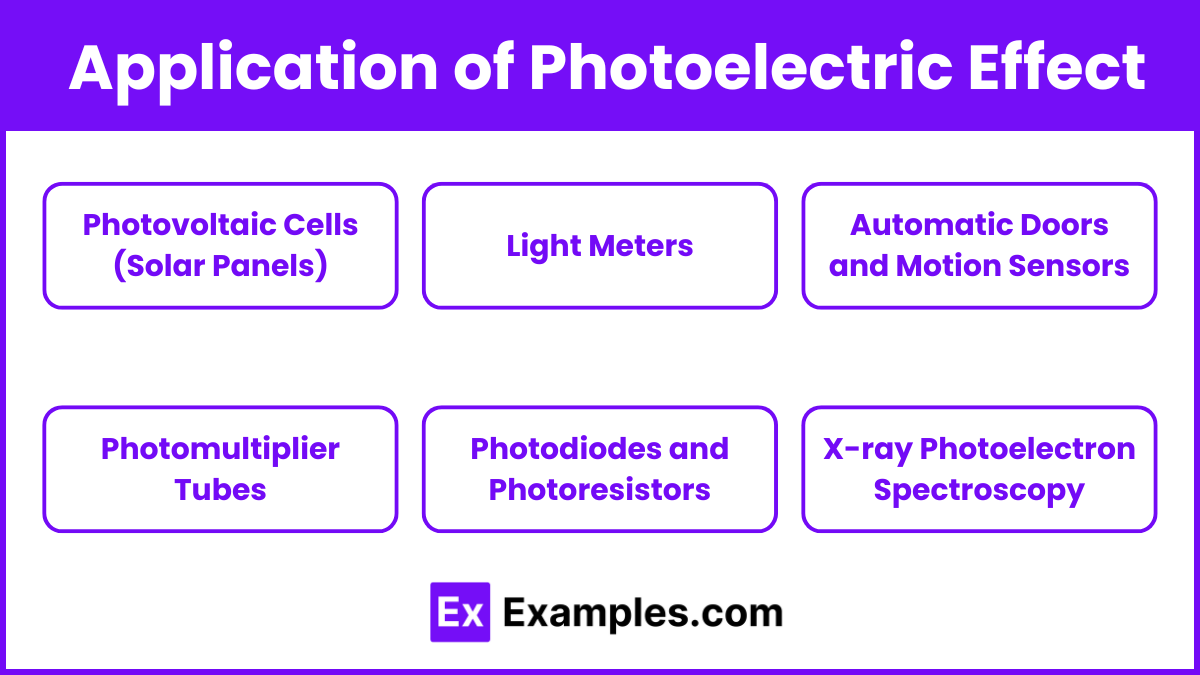
- Photovoltaic Cells (Solar Panels)
- Energy Conversion: Photovoltaic cells use the photoelectric effect to convert sunlight directly into electricity. When photons strike the semiconductor material in the cell, they emit electrons, creating an electric current.
- Renewable Energy: This technology is fundamental in solar panels, providing a sustainable and renewable source of energy.
- Light Meters
- Photography: Light meters measure the intensity of light and are crucial in photography for setting the correct exposure. These devices rely on the photoelectric effect to measure the amount of light hitting a sensor.
- Illumination Measurement: They are also used in various industries to measure light levels for proper illumination and safety standards.
- Automatic Doors and Motion Sensors
- Detection Systems: Automatic doors use sensors that detect changes in light intensity caused by the presence of a person. When someone approaches, the change in light triggers the photoelectric effect, activating the door mechanism.
- Security Systems: Motion sensors in security systems also rely on the photoelectric effect to detect intruders by sensing changes in light patterns.
- Photomultiplier Tubes
- Radiation Detection: Photomultiplier tubes are highly sensitive detectors of light and radiation. They amplify the photoelectric effect, making them useful in applications ranging from medical imaging to scientific research.
- Astronomy: These tubes are also used in telescopes and other astronomical instruments to detect faint light from distant stars and galaxies.
- Photodiodes and Photoresistors
- Electronic Devices: Photodiodes and photoresistors are used in various electronic devices to detect light levels. They are critical components in optical communication systems, remote controls, and light-sensitive circuits.
- Automatic Lighting: These components are also used in automatic lighting systems, where lights turn on or off based on the detected light intensity.
- X-ray Photoelectron Spectroscopy (XPS)
- Surface Analysis: XPS uses the photoelectric effect to analyze the surface chemistry of materials. By measuring the kinetic energy of emitted electrons, scientists can determine the elemental composition and chemical state of a material’s surface.
- Material Science: This technique is essential in material science, nanotechnology, and surface engineering for developing new materials and coatings.
Examples Problems
- Solar Panels: Convert sunlight into electricity using photovoltaic cells, generating an electric current when photons cause electron emission.
- Digital Cameras: Use light sensors to capture images by converting emitted electrons into an electronic signal.
- Automatic Doors: Sensors detect changes in light intensity, triggering the door to open when someone approaches.
- Smoke Detectors: Photoelectric smoke detectors set off alarms when smoke scatters light and triggers the photoelectric effect.
- Television Remote Controls: Use infrared light to transmit signals, detected and converted into electronic commands by the receiver.
- Photomultiplier Tubes: Amplify weak light signals by converting photons into electrons and multiplying them for a stronger signal.
- X-ray Photoelectron Spectroscopy (XPS): Analyzes surface chemistry by measuring the kinetic energy of electrons emitted by X-rays.
- Light Sensors in Smartphones: Adjust screen brightness based on ambient light using the photoelectric effect to detect changes in light intensity.