Alpha-carbon chemistry refers to the study of reactions involving the carbon atom directly adjacent to a carbonyl group (known as the alpha-carbon). This chemistry is crucial because the alpha-hydrogens are acidic due to resonance stabilization by the carbonyl group, making them more prone to deprotonation. As a result, the alpha-carbon can form enolate ions, which act as nucleophiles in many reactions like aldol condensation, enolate alkylation, and Michael addition. These reactions are fundamental in organic synthesis, enabling the construction of complex molecules and diverse chemical structures.
Learning Objectives
In studying “Alpha-Carbon Chemistry” for the MCAT, focus on mastering the reactivity of alpha-hydrogens and the conditions that lead to enolate formation. Start by reviewing the acidity of alpha-hydrogens and the stabilization of enolates through resonance with the carbonyl group. Dive into key reactions like aldol condensation, Claisen condensation, and Michael addition, analyzing their mechanisms step-by-step. Practice applying these reactions to both synthetic organic compounds and metabolic processes, such as the citric acid cycle and gluconeogenesis. Solve reaction mechanism problems to enhance your ability to predict products, intermediates, and reaction conditions. This will strengthen your conceptual understanding and problem-solving skills in alpha-carbon chemistry.
Key Concepts of Alpha-Carbon Chemistry
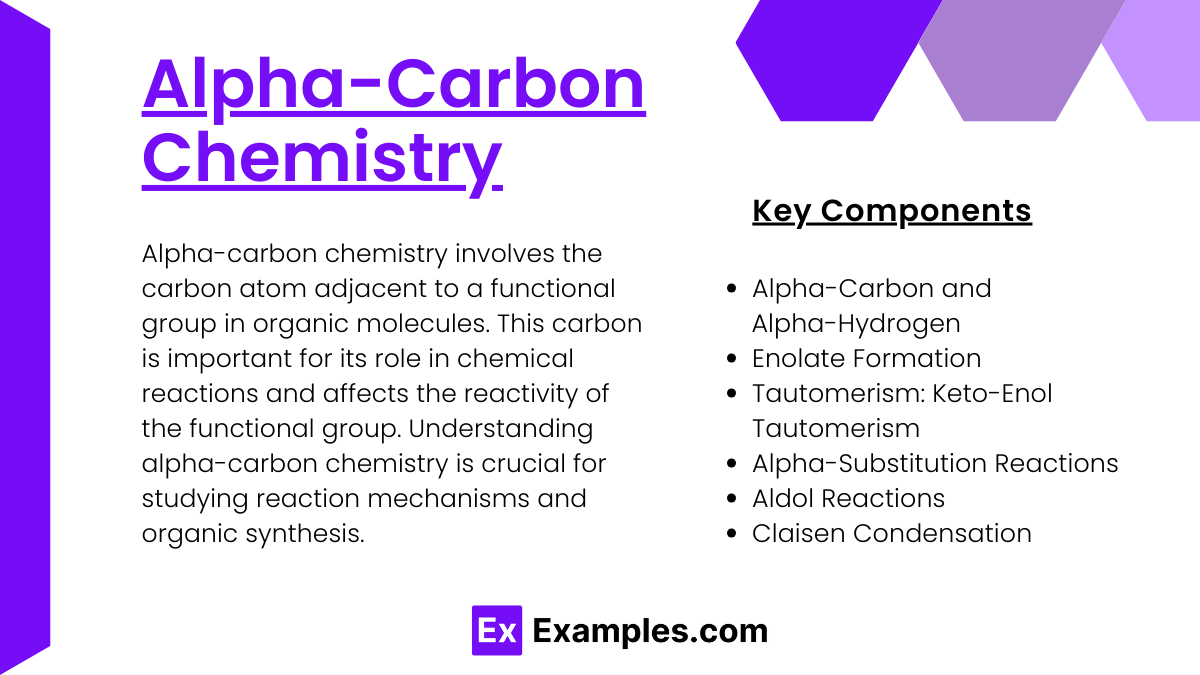
1. Alpha-Carbon and Alpha-Hydrogen
- The alpha-carbon is the carbon directly adjacent to the carbonyl carbon in carbonyl compounds.
- Alpha-hydrogens are acidic due to the resonance stabilization of the resulting enolate ion after deprotonation.
- These alpha-hydrogens are easily removed by bases, making them central to several key organic reactions.
2. Enolate Formation
- An enolate is formed when a base deprotonates an alpha-hydrogen of a carbonyl compound.
- Common bases used: LDA (lithium diisopropylamide), NaOH, and KOH.
- Enolates are nucleophilic and participate in carbon-carbon bond-forming reactions.
3. Tautomerism: Keto-Enol Tautomerism
- Tautomerism is an equilibrium between the keto form (carbonyl) and the enol form (contains an -OH group).
- This process is catalyzed by both acids and bases and involves the transfer of an alpha-hydrogen.
4. Alpha-Substitution Reactions
- Alpha-substitution involves the replacement of an alpha-hydrogen with another group.
- Common reactions:
- Halogenation: Under acidic or basic conditions, alpha-hydrogens can be replaced with halogens (Cl, Br, I).
- Alkylation: Enolates can be alkylated by reacting with alkyl halides, introducing new carbon chains.
5. Aldol Reactions
- Aldol Addition: Enolate ions attack another carbonyl compound, forming a β-hydroxy carbonyl.
- Aldol Condensation: Heating leads to the loss of water, producing α,β-unsaturated carbonyl compounds.
6. Claisen Condensation
- Similar to the aldol reaction but occurs between esters.
- Results in β-keto esters or β-diketones, depending on the reactants used.
Important Reactions in Alpha-Carbon Chemistry
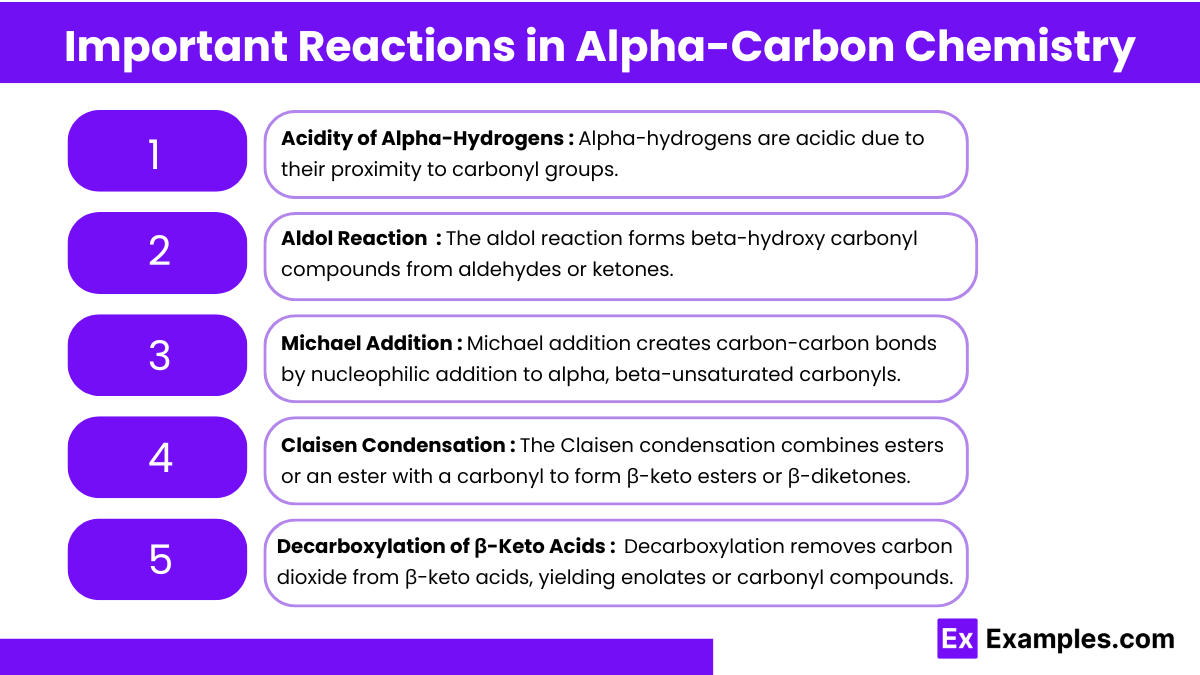
1. Acidity of Alpha-Hydrogens
- Alpha-hydrogens in carbonyl compounds have a lower pKa, making them more acidic.
- The enolate ion formed after deprotonation is stabilized by resonance.
2. Aldol Reaction (Aldol Addition & Condensation)
- Aldol Addition: Forms a β-hydroxy carbonyl by adding the enolate to another aldehyde or ketone.
- Aldol Condensation: Heating removes water, forming α,β-unsaturated carbonyl.
3. Michael Addition
- A Michael addition involves the nucleophilic addition of an enolate to an α,β-unsaturated carbonyl compound.
- This reaction is used in complex molecule synthesis.
4. Claisen Condensation
- Involves two esters or an ester and a ketone forming a β-keto ester.
- Requires strong bases like NaOEt or NaH.
5. Decarboxylation of β-Keto Acids
- Decarboxylation occurs when β-keto acids are heated, releasing CO₂ and forming ketones.
- This reaction is common in metabolic pathways.
Tips for Alpha-Carbon Chemistry
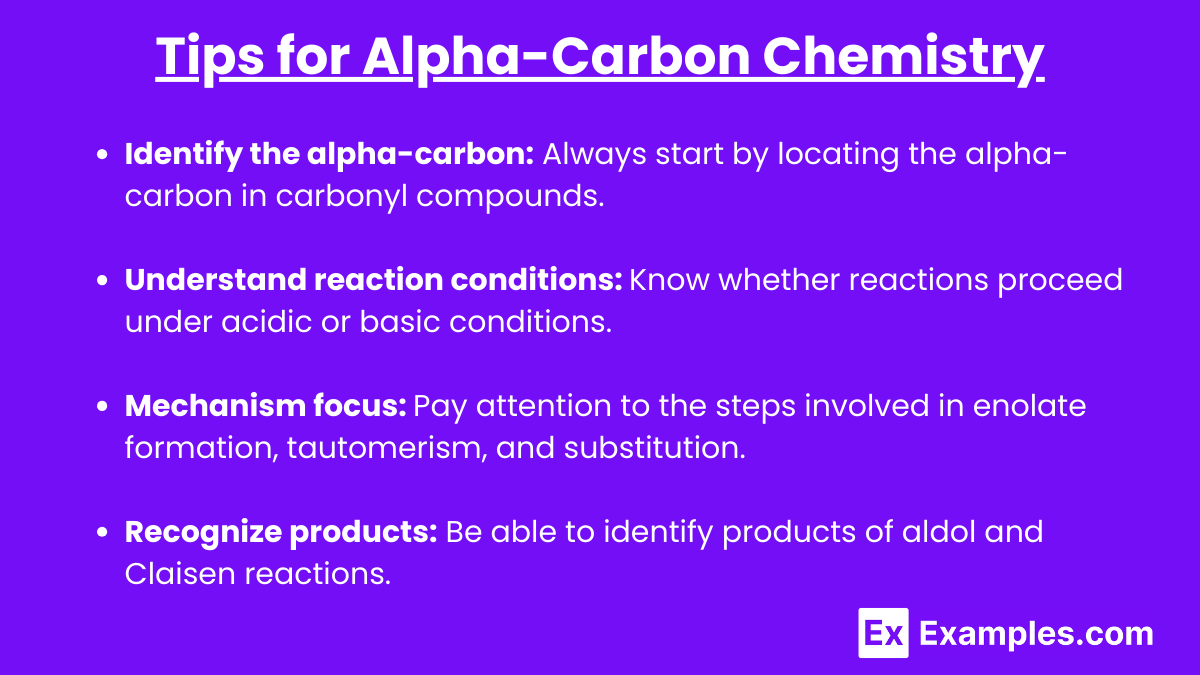
- Identify the alpha-carbon: Always start by locating the alpha-carbon in carbonyl compounds.
- Understand reaction conditions: Know whether reactions proceed under acidic or basic conditions.
- Mechanism focus: Pay attention to the steps involved in enolate formation, tautomerism, and substitution.
- Recognize products: Be able to identify products of aldol and Claisen reactions.
- Biological relevance: Relate these reactions to metabolic pathways like glycolysis, gluconeogenesis, and fatty acid synthesis.
Examples
Example 1. Enzyme-Catalyzed Reactions in Metabolism
In metabolic pathways, enzymes often catalyze reactions involving alpha-carbon chemistry. For instance, transamination reactions convert amino acids into corresponding alpha-keto acids, which play a central role in energy production and biosynthesis. These reactions are pivotal in the Krebs cycle, where the alpha-carbon of amino acids like glutamate is targeted by transaminases to facilitate the conversion to intermediates like alpha-ketoglutarate.
Example 2. Alpha-Carboxylation in Biosynthesis
Alpha-carbon chemistry is essential in biosynthetic processes, such as fatty acid synthesis. During this process, acetyl-CoA molecules undergo carboxylation at the alpha-carbon to form malonyl-CoA, which serves as a building block for longer fatty acid chains. This modification at the alpha-carbon is crucial for chain elongation, impacting the formation of lipids that are vital for cell membrane structure and energy storage.
Example 3. Alpha-Carbon Reactions in Drug Synthesis
In pharmaceutical chemistry, alpha-carbon modifications are often performed to enhance drug activity or bioavailability. For example, alpha-halogenation is a common strategy used to make compounds more reactive for subsequent transformations. By selectively introducing functional groups at the alpha-carbon, medicinal chemists can create analogs of natural compounds that have improved efficacy or reduced side effects.
Example 4. Enolate Formation in Organic Synthesis
Enolate formation is a classic example of alpha-carbon chemistry in organic synthesis. This reaction occurs when a base abstracts a proton from the alpha-carbon of a carbonyl compound, generating an enolate anion. The enolate then acts as a nucleophile, enabling the synthesis of new carbon-carbon bonds in processes like aldol reactions, Claisen condensations, and Michael additions, which are foundational techniques in chemical synthesis.
Example 5. Alpha-Deprotonation in Peptide Synthesis
In peptide synthesis, alpha-carbon chemistry is essential during the formation of peptide bonds. For instance, alpha-deprotonation of amino acids is a key step that allows for the coupling of amino acid residues. By activating the alpha-carbon, chemists facilitate the nucleophilic attack necessary to form amide bonds, which are the backbone of proteins and peptides used in research, pharmaceuticals, and biotechnology.
Practice Questions
Question 1
Which of the following conditions is most likely to favor enolate formation from a ketone?
- A) Basic and low temperature
- B) Acidic and low temperature
- C) Neutral pH and high temperature
- D) Acidic and high temperature
Answer: A) Basic and low temperature
Explanation: Enolates are formed when a base abstracts an alpha-hydrogen from a ketone, generating a negatively charged enolate ion. Strong bases (e.g., LDA or NaH) are typically used to drive this reaction because they are capable of deprotonating the relatively acidic alpha-hydrogen. The process is often carried out at low temperatures to prevent unwanted side reactions, like aldol condensation or further enolate formation. Acidic conditions do not favor enolate formation as they lead to the formation of enols, which are less reactive than enolates.
Question 2
Which of the following statements about alpha-carbon acidity is true?
- A) The alpha-hydrogens of aldehydes are less acidic than those of ketones.
- B) The alpha-hydrogens of esters are more acidic than those of aldehydes.
- C) The alpha-hydrogens of amides are less acidic than those of esters.
- D) The alpha-hydrogens of nitriles are less acidic than those of ketones.
Answer: C) The alpha-hydrogens of amides are less acidic than those of esters.
Explanation: The acidity of alpha-hydrogens is influenced by the ability of the adjacent functional group to stabilize the resulting negative charge after deprotonation. Esters have more electron-withdrawing ability than amides, making the alpha-hydrogens of esters more acidic. Aldehydes generally have more acidic alpha-hydrogens than ketones because the aldehyde’s carbonyl carbon is more electrophilic, stabilizing the negative charge better. Nitriles have highly acidic alpha-hydrogens due to the strong electron-withdrawing effect of the cyano group, making them more acidic than ketones.
Question 3
In the aldol reaction, which step involves the attack of an enolate on a carbonyl carbon?
- A) Enolate formation
- B) Carbonyl activation
- C) Nucleophilic addition
- D) Aldol dehydration
Answer: C) Nucleophilic addition
Explanation: In the aldol reaction, the enolate acts as a nucleophile and attacks the carbonyl carbon of another molecule, leading to the formation of a beta-hydroxy carbonyl compound (aldol). This step is specifically called nucleophilic addition because the negatively charged enolate carbon adds to the partially positive carbonyl carbon, forming a new carbon-carbon bond. Enolate formation is the first step, where the base abstracts an alpha-hydrogen. Aldol dehydration is the final step, where the beta-hydroxy carbonyl compound loses water to form an alpha, beta-unsaturated carbonyl compound.