Bioenergetics is essential to biological systems, focusing on the energy flow through cells and organisms. On the MCAT, you’ll explore concepts such as thermodynamics, ATP production, and metabolic pathways. Understanding cellular respiration, photosynthesis, and the role of enzymes in energy transfer is crucial, as these topics are fundamental to mastering biochemistry and physiology sections of the exam.
Learning Objectives
In studying “Bioenergetics” for the MCAT, you should learn to understand how energy is produced, transferred, and utilized in biological systems. Analyze the role of ATP as the primary energy currency and how it is generated through metabolic pathways, including glycolysis, the citric acid cycle, and oxidative phosphorylation. Evaluate the role of enzymes and coupled reactions in facilitating energy flow. Understand the thermodynamic principles governing bioenergetic processes, such as Gibbs free energy and entropy, and how they relate to spontaneity in biological reactions. Additionally, explore how disruptions in energy metabolism affect cellular function and contribute to disease states, preparing you to apply these principles to biochemical and physiological contexts on the MCAT.
Understanding Energy Production, Transfer, and Utilization in Biological Systems
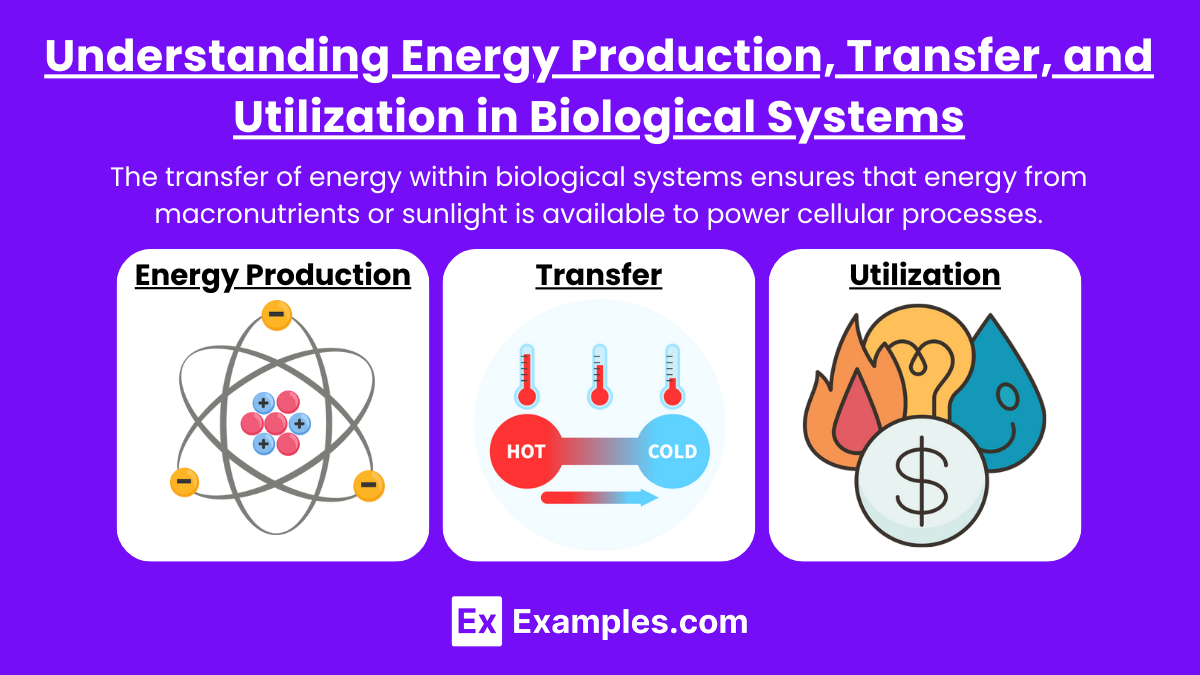
1. Energy Production in Biological Systems
Energy production primarily occurs through cellular respiration and photosynthesis.
a. Cellular Respiration: In eukaryotic orgUnderstanding Energy Production, Transfer, and Utilization in Biological Systemsanisms, energy is produced by breaking down glucose (or other macronutrients) in a multi-step process, releasing energy to form adenosine triphosphate (ATP).
Stages of Cellular Respiration:
- Glycolysis (cytoplasm): Glucose is converted into pyruvate, producing a small amount of ATP and NADH.
- Krebs Cycle (mitochondria): Pyruvate is further broken down, releasing carbon dioxide and generating NADH and FADH₂.
- Electron Transport Chain (ETC) (inner mitochondrial membrane): Electrons from NADH and FADH₂ flow through the ETC, creating a proton gradient that drives ATP synthesis.
- Net Energy Production: From one molecule of glucose, approximately 36–38 ATP molecules are generated.
b. Photosynthesis:
- In plants and some microorganisms, energy is produced by converting light energy into chemical energy, stored in glucose.
- Light-dependent reactions (chloroplasts) capture light energy to produce ATP and NADPH.
- Calvin Cycle uses ATP and NADPH to convert carbon dioxide into glucose, which can later be used in respiration.
2. Energy Transfer in Biological Systems
The transfer of energy within biological systems ensures that energy from macronutrients or sunlight is available to power cellular processes.
a. ATP as the Energy Currency:
- ATP stores energy in its high-energy phosphate bonds, which can be readily broken to release energy for cellular activities.
ATP→ADP+P+Energy - ATP powers activities such as muscle contraction, active transport across membranes, and enzyme-catalyzed reactions.
b. Electron Carriers:
- NADH, FADH₂, and NADPH act as electron carriers, transferring high-energy electrons to metabolic pathways.
- In respiration, electrons transferred through the ETC generate a proton gradient that powers ATP production.
3. Utilization of Energy in Biological Systems
Organisms use energy to perform essential functions that maintain life.
a. Cellular Work:
- Mechanical Work: Muscle contraction and intracellular transport of molecules.
- Chemical Work: Synthesis of macromolecules (proteins, DNA) for growth and repair.
- Transport Work: Active transport of ions and molecules across cell membranes, maintaining concentration gradients (e.g., sodium-potassium pump).
b. Metabolic Pathways:
- Catabolic Pathways: Breakdown of molecules (e.g., glycolysis, Krebs cycle) to release energy.
- Anabolic Pathways: Synthesis of complex molecules (e.g., protein synthesis) using energy from ATP.
c. Thermoregulation:
- Energy is used to maintain a stable body temperature in endothermic organisms through processes like shivering and sweating.
ATP: The Primary Energy Currency of Cells
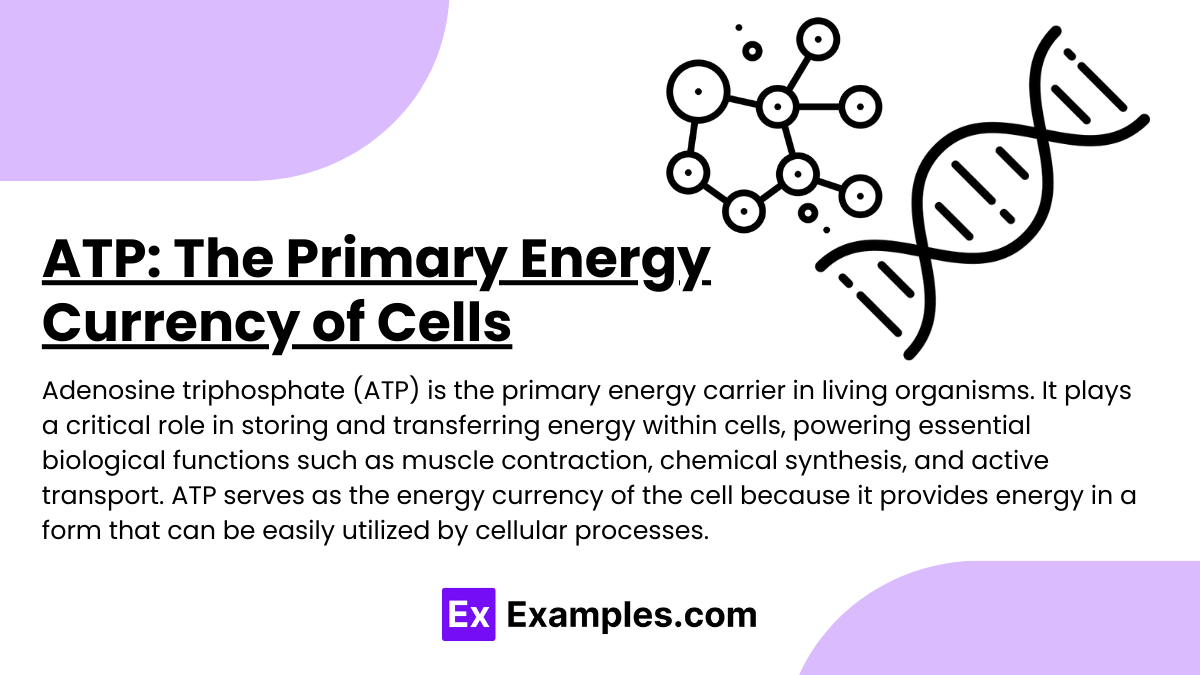
Adenosine triphosphate (ATP) is the primary energy carrier in living organisms. It plays a critical role in storing and transferring energy within cells, powering essential biological functions such as muscle contraction, chemical synthesis, and active transport. ATP serves as the energy currency of the cell because it provides energy in a form that can be easily utilized by cellular processes.
Structure of ATP
ATP consists of three key components:
- Adenine: A nitrogenous base.
- Ribose: A five-carbon sugar.
- Three Phosphate Groups: Linked in a chain, with high-energy bonds between the phosphate groups.
- The energy stored in ATP is mainly concentrated in the bonds between the second and third phosphate groups. When these bonds are broken, energy is released to power cellular activities.
How ATP Provides Energy
ATP provides energy by hydrolyzing (breaking) the bond between the second and third phosphate groups, forming adenosine diphosphate (ADP) and an inorganic phosphate (Pi):
ATP → ADP+Pi+Energy
This reaction releases energy that can be used to perform work inside the cell.
Regeneration of ATP
Cells continuously regenerate ATP from ADP through various metabolic pathways, ensuring a steady energy supply:
- Cellular Respiration: ATP is synthesized from glucose breakdown in the mitochondria through glycolysis, the Krebs cycle, and oxidative phosphorylation.
- Photosynthesis (in plants): ATP is produced during the light-dependent reactions to power the Calvin cycle.
- Fermentation: In anaerobic conditions, ATP is produced through glycolysis, although in smaller amounts.
Functions of ATP in Cells
- Mechanical Work: Powers muscle contraction and intracellular transport of molecules via motor proteins (e.g., actin and myosin).
- Chemical Work: Provides energy for biosynthesis of macromolecules like proteins, nucleic acids, and carbohydrates.
- Transport Work: Drives active transport of ions and molecules across cell membranes (e.g., sodium-potassium pump), maintaining concentration gradients.
- Signal Transduction: ATP is involved in cell signaling, such as phosphorylation cascades, which regulate enzyme activity and cellular responses.
- Heat Production: ATP hydrolysis releases energy, some of which is dissipated as heat, helping maintain body temperature in warm-blooded organisms.
Role of Enzymes and Coupled Reactions in Energy Flow
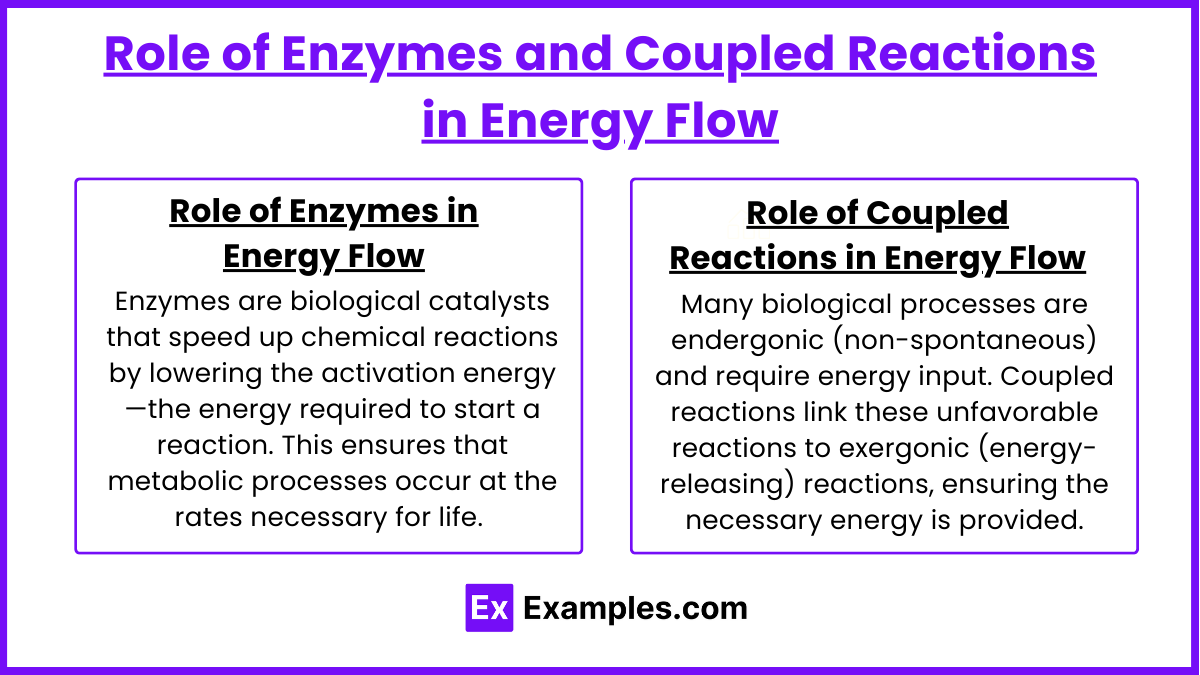
In biological systems, enzymes and coupled reactions are essential for efficient energy flow, enabling cells to drive chemical reactions necessary for life. Enzymes accelerate the rate of biochemical reactions, while coupled reactions allow energy from one reaction to power another, ensuring energy is used efficiently.
Role of Enzymes in Energy Flow
Enzymes are biological catalysts that speed up chemical reactions by lowering the activation energy—the energy required to start a reaction. This ensures that metabolic processes occur at the rates necessary for life.
How Enzymes Work
- Substrate Binding: The enzyme binds to a specific reactant (substrate) at the active site.
- Formation of the Enzyme-Substrate Complex: The enzyme stabilizes the transition state, making it easier for the substrate to be converted into products.
- Product Release: After the reaction, the products are released, and the enzyme remains unchanged, ready to catalyze another reaction.
Enzyme Specificity and Regulation
- Specificity: Each enzyme is specific to a particular substrate or reaction.
- Regulation: Enzyme activity is regulated through allosteric interactions, feedback inhibition, and covalent modifications (e.g., phosphorylation), ensuring that energy production and consumption are balanced.
Examples of Enzyme Activity in Energy Flow
- ATP Synthase: This enzyme produces ATP during cellular respiration by utilizing the proton gradient in mitochondria.
- Hexokinase: Catalyzes the first step in glycolysis, converting glucose into glucose-6-phosphate, initiating the process of ATP production.
Role of Coupled Reactions in Energy Flow
Many biological processes are endergonic (non-spontaneous) and require energy input. Coupled reactions link these unfavorable reactions to exergonic (energy-releasing) reactions, ensuring the necessary energy is provided.
ATP and Coupled Reactions
- ATP Hydrolysis is a common exergonic reaction used to drive endergonic processes: ATP→ADP+Pi+Energy
- The energy released from ATP hydrolysis is transferred to power processes like muscle contraction, biosynthesis, and active transport.
Examples of Coupled Reactions in Energy Flow
- Active Transport: The sodium-potassium pump uses ATP to move sodium and potassium ions against their concentration gradients, maintaining cellular ion balance.
- Muscle Contraction: ATP hydrolysis provides the energy needed for the interaction of actin and myosin filaments, enabling muscle movement.
- Biosynthesis of Macromolecules: The synthesis of complex molecules like proteins and nucleic acids requires energy from ATP hydrolysis to link monomers into polymers.
- Cellular Respiration and ATP Production: In oxidative phosphorylation, the flow of electrons through the electron transport chain is coupled to the synthesis of ATP using a proton gradient.
Impact of Disruptions in Energy Metabolism on Cellular Function and Disease
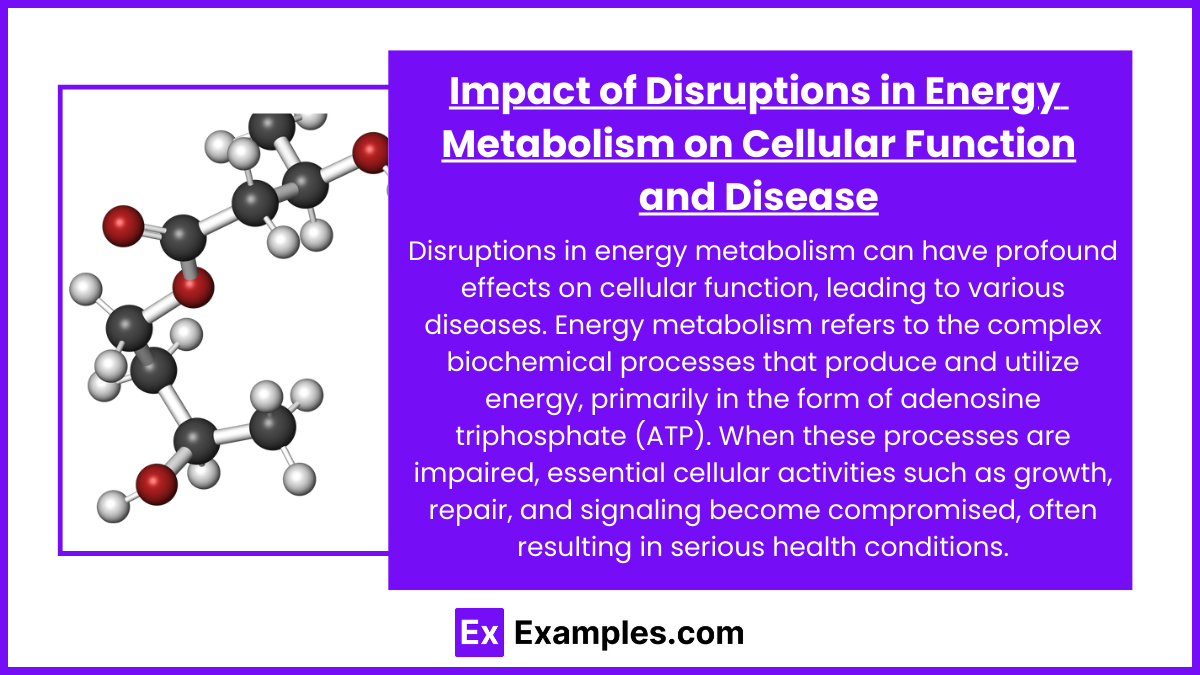
Disruptions in energy metabolism can have profound effects on cellular function, leading to various diseases. Energy metabolism refers to the complex biochemical processes that produce and utilize energy, primarily in the form of adenosine triphosphate (ATP). When these processes are impaired, essential cellular activities such as growth, repair, and signaling become compromised, often resulting in serious health conditions.
Processes in Energy Metabolism
- Cellular Respiration: The breakdown of glucose, fats, or proteins to produce ATP through glycolysis, the Krebs cycle, and oxidative phosphorylation.
- ATP Production: ATP powers essential cellular processes such as muscle contraction, active transport, and protein synthesis.
- Mitochondrial Function: The mitochondria are the powerhouse of the cell, where most ATP is generated through oxidative phosphorylation.
Disruptions in these processes reduce energy availability, impair cellular function, and trigger disease states.
Causes of Disruptions in Energy Metabolism
- Genetic Mutations:
- Mutations in mitochondrial DNA or nuclear genes that affect enzymes in energy production pathways can impair ATP synthesis.
- Example: Mitochondrial diseases such as Leber’s hereditary optic neuropathy result from defective electron transport chain proteins.
- Nutritional Deficiencies:
- Inadequate glucose or essential vitamins (such as vitamin B1 or B12) impairs cellular respiration and ATP production.
- Example: Beriberi, caused by thiamine deficiency, affects ATP synthesis and disrupts muscle and nerve function.
- Hypoxia (Oxygen Deficiency):
- Lack of oxygen reduces oxidative phosphorylation, shifting cells to less efficient anaerobic glycolysis.
- Example: Ischemia during a heart attack or stroke deprives tissues of oxygen, leading to cell death.
- Toxins and Drugs:
- Substances like cyanide inhibit cytochrome enzymes in the electron transport chain, halting ATP production.
- Certain chemotherapeutic agents impair mitochondrial function, causing muscle fatigue and weakness.
- Hormonal Imbalance: Conditions like diabetes mellitus disrupt glucose metabolism, reducing ATP availability and impairing cellular function.
Diseases Associated with Energy Metabolism Disruption
- Mitochondrial Disorders: Inherited mitochondrial diseases impair ATP production, leading to fatigue, muscle weakness, and neurodegeneration.
- Type 2 Diabetes Mellitus: Impaired glucose metabolism results in reduced ATP production and oxidative stress, leading to tissue damage and complications like neuropathy and kidney failure.
- Neurodegenerative Diseases: Diseases such as Parkinson’s and Alzheimer’s involve mitochondrial dysfunction, leading to oxidative stress, cell death, and cognitive decline.
- Cancer: Cancer cells exhibit altered metabolism (the Warburg effect), favoring glycolysis even in the presence of oxygen. This shift in metabolism supports rapid growth but creates vulnerabilities that can be targeted therapeutically.
- Cardiovascular Diseases: In conditions like heart failure and ischemia, impaired energy metabolism reduces the ability of heart muscle cells to contract effectively, leading to organ dysfunction.
Examples
Example 1: Cellular Respiration
Cellular respiration is a fundamental bioenergetic process where cells convert glucose and oxygen into energy in the form of ATP. This multi-step process includes glycolysis, the Krebs cycle, and the electron transport chain. During cellular respiration, energy stored in organic molecules is transformed into ATP, which powers various cellular activities, making it essential for life.
Example 2: Photosynthesis
In plants, photosynthesis is a vital bioenergetic process that converts light energy from the sun into chemical energy stored in glucose. Chloroplasts capture sunlight, which drives the conversion of carbon dioxide and water into glucose and oxygen. This process not only fuels plant growth but also provides energy for other organisms through the food chain, illustrating the interconnectedness of bioenergetics in ecosystems.
Example 3: ATP Synthesis in Mitochondria
The mitochondria, often referred to as the “powerhouses” of the cell, play a crucial role in bioenergetics by synthesizing ATP. The process of oxidative phosphorylation occurs in the inner mitochondrial membrane, where the electron transport chain generates a proton gradient that drives ATP synthesis through ATP synthase. This mechanism is fundamental to energy production in aerobic organisms.
Example 4: Muscle Contraction
Bioenergetics is essential in muscle contraction, where ATP provides the energy required for muscle fibers to shorten and generate force. During physical activity, the stored ATP in muscles is quickly consumed, and additional ATP is generated through cellular respiration and anaerobic processes, such as lactic acid fermentation. This example highlights the immediate energy needs of muscles and the dynamic nature of bioenergetics in response to physical demands.
Example 5: Metabolic Pathways
Various metabolic pathways, such as glycolysis and the citric acid cycle, illustrate the principles of bioenergetics by converting nutrients into usable energy. For instance, glycolysis breaks down glucose into pyruvate, releasing energy that is captured as ATP. These pathways are interconnected, and the regulation of energy flow through these metabolic routes is critical for maintaining cellular homeostasis and supporting life processes.
Practice Questions
Question 1
What is the primary function of ATP (adenosine triphosphate) in biological systems?
A) To serve as a genetic material
B) To store and transfer energy
C) To transport nutrients across cell membranes
D) To catalyze biochemical reactions
Correct Answer: B) To store and transfer energy.
Explanation: ATP is known as the “energy currency” of the cell. Its primary role is to store and transfer energy for various cellular processes. When ATP is hydrolyzed, it releases energy that can be used for activities such as muscle contraction, active transport, and biochemical reactions. Options A, C, and D describe other functions of biological molecules, but they do not accurately reflect the primary role of ATP in bioenergetics.
Question 2
Which of the following processes is an example of bioenergetics in action?
A) DNA replication
B) Protein synthesis
C) Cellular respiration
D) Osmosis
Correct Answer: C) Cellular respiration.
Explanation: Cellular respiration is a key process in bioenergetics where cells convert glucose and oxygen into energy in the form of ATP. This process involves multiple steps, including glycolysis, the Krebs cycle, and the electron transport chain. It illustrates how energy stored in organic molecules is transformed into usable energy for the cell. Options A and B are vital cellular processes, but they do not directly involve energy transformation in the same way that cellular respiration does. Option D, osmosis, refers to the movement of water across a membrane and does not involve energy transformation.
Question 3
In the context of bioenergetics, what role do enzymes play?
A) They provide energy directly to the cell.
B) They lower the activation energy of biochemical reactions.
C) They store genetic information.
D) They transport molecules across cell membranes.
Correct Answer: B) They lower the activation energy of biochemical reactions.
Explanation: Enzymes are biological catalysts that speed up biochemical reactions by lowering the activation energy required for the reaction to proceed. This allows metabolic processes to occur more efficiently and at lower temperatures, facilitating the flow of energy within the cell. While enzymes do not provide energy directly or store genetic information, they play a crucial role in bioenergetics by enabling the transformations of energy through metabolic pathways. Options C and D describe other functions of biological molecules, but they do not pertain to the primary function of enzymes in bioenergetics.