Capacitors are fundamental components in electrical circuits, essential for storing and releasing electrical energy. On the MCAT, you’ll explore the principles of capacitance, dielectric materials, and the behavior of capacitors in series and parallel arrangements. Understanding energy storage, charging and discharging processes, and applications in circuits is crucial, as these concepts are key to mastering the physics and engineering sections of the exam.
Learning Objectives
In studying “Capacitors” for the MCAT, you should learn to understand the function of capacitors in storing electrical energy and charge. Identify key properties such as capacitance, dielectric materials, and how capacitance changes with geometry and material composition. Analyze the behavior of capacitors in series and parallel circuits and how they affect total capacitance. Understand the role of electric fields in capacitors and the energy stored in these fields. Apply formulas for capacitance, charge, voltage, and energy to solve physics-based MCAT problems. Additionally, explore practical applications of capacitors in biological systems, such as in cell membranes, and in medical devices like defibrillators.
Understanding the Function of Capacitors
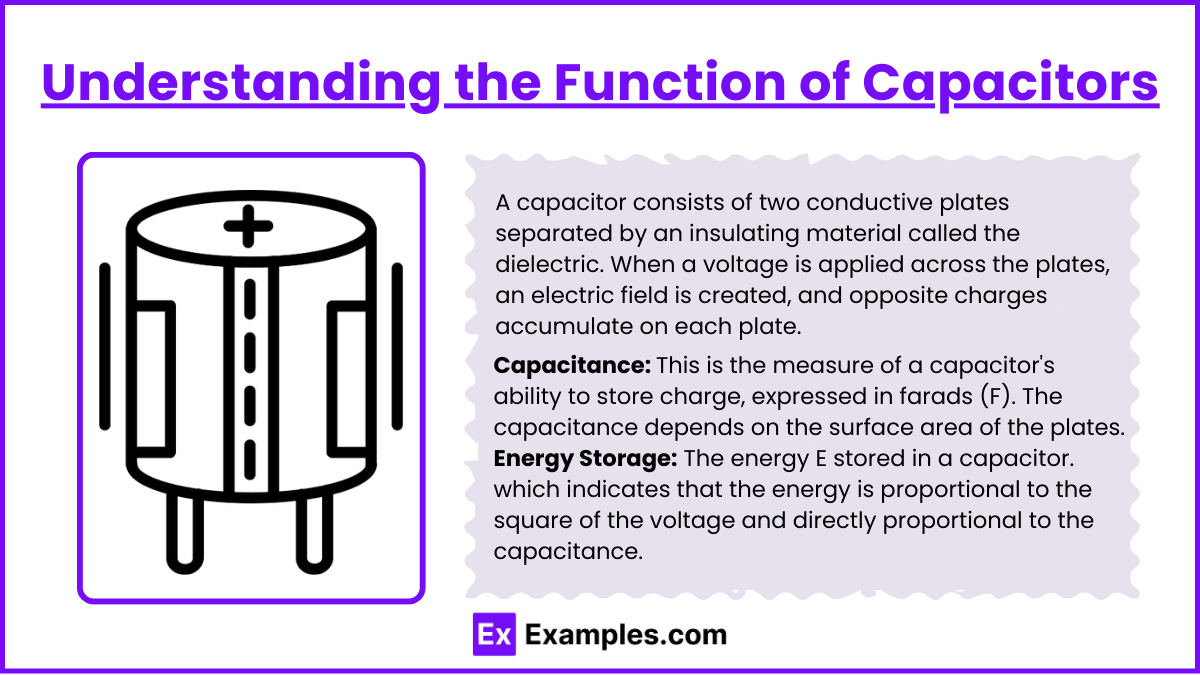
Basic Construction and Operation
A capacitor consists of two conductive plates separated by an insulating material called the dielectric. When a voltage is applied across the plates, an electric field is created, and opposite charges accumulate on each plate, storing electrical energy in the form of an electrostatic field.
Key Characteristics:
- Capacitance: This is the measure of a capacitor’s ability to store charge, expressed in farads (F). The capacitance C is given by: C = Q/V where Q is the charge stored, and V is the voltage across the capacitor. The capacitance depends on the surface area of the plates, the distance between them, and the properties of the dielectric material.
- Energy Storage: The energy E stored in a capacitor is given by: E = 1/2CV2 which indicates that the energy is proportional to the square of the voltage and directly proportional to the capacitance.
Electric Fields and Energy Storage in Capacitors
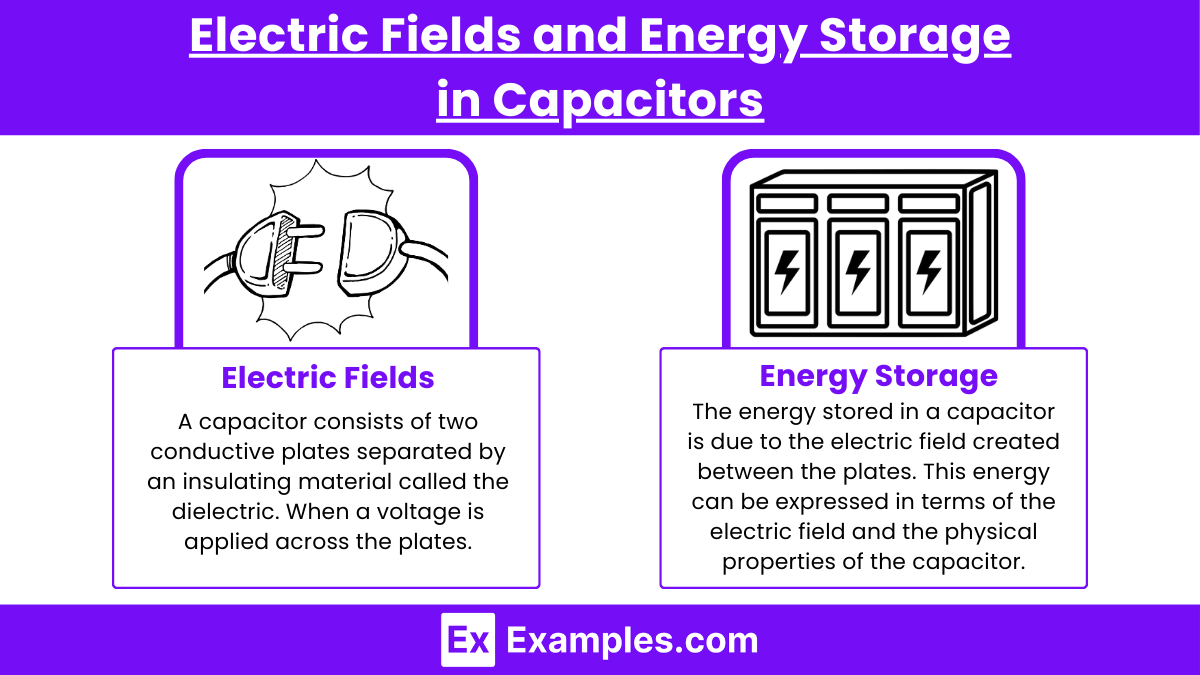
Electric Fields in Capacitors
A capacitor consists of two conductive plates separated by an insulating material known as a dielectric. When a voltage is applied across these plates, an electric field is established between them, causing positive charge to accumulate on one plate and negative charge on the opposite plate.
Key Aspects:
- Electric Field Creation: The electric field (E) in a capacitor is essentially uniform between the plates and is directed from the positive to the negative plate. The strength of this field is directly proportional to the amount of charge on the plates and inversely proportional to the separation between them.
- Dielectric Material: The dielectric material between the plates increases the capacitor’s ability to store charge at a given voltage by reducing the electric field’s effect within the material itself. This reduction is quantified by the dielectric constant (κ), which is a measure of how effectively a material can reduce the field compared to vacuum.
The electric field E between the plates of a parallel plate capacitor can be expressed as: E = V/d where V is the voltage across the capacitor, and d is the distance between the plates.
Energy Storage in Capacitors
The energy stored in a capacitor is due to the electric field created between the plates. This energy can be expressed in terms of the electric field and the physical properties of the capacitor:
Energy Storage Formula:
- The energy (U) stored in a capacitor is given by: U = 1/2CV2 where C is the capacitance, and V is the voltage across the plates.
Energy in Terms of Electric Field:
- Alternatively, the energy can also be expressed in terms of the electric field and the geometry of the capacitor. For a parallel plate capacitor, the energy stored can be written as: U = 1/2ϵ0ϵrE2Ad where ϵ0 is the permittivity of free space, ϵr is the relative permittivity of the dielectric, E is the electric field, A is the area of one plate, and d is the separation between the plates.
Practical Applications of Capacitors in Biological and Medical Systems
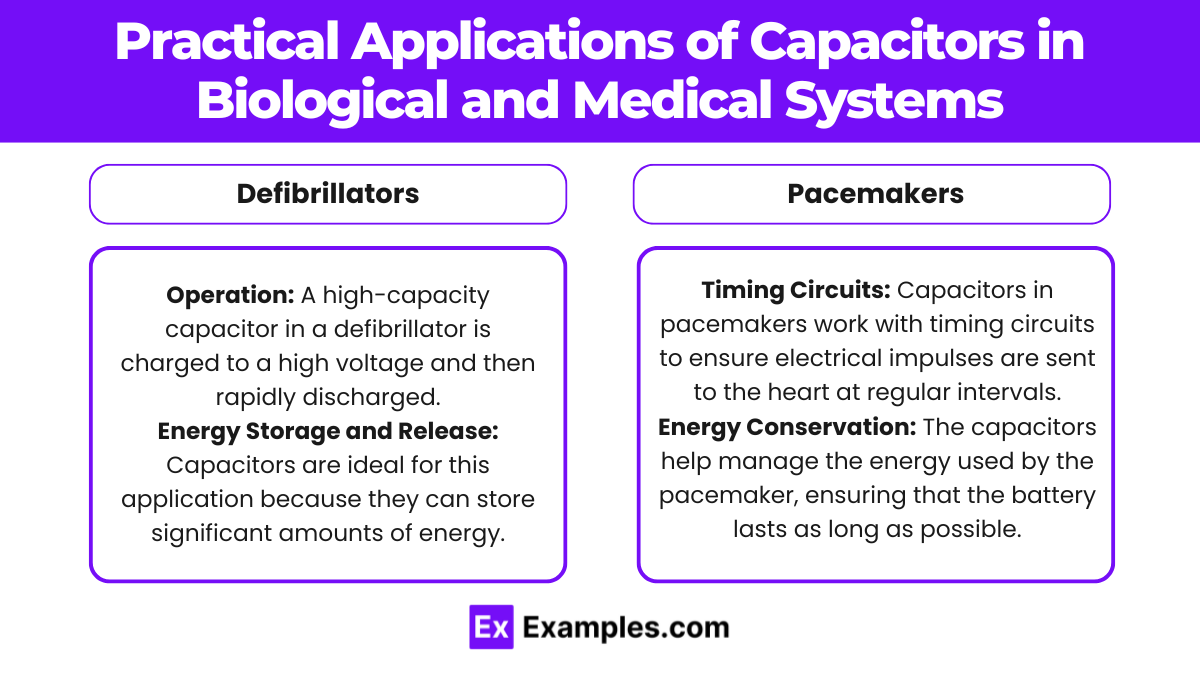
Defibrillators
One of the most critical medical uses of capacitors is in defibrillators. These devices are designed to deliver a controlled electric shock to the heart when it is experiencing arrhythmia, such as ventricular fibrillation or tachycardia.
- Operation: A high-capacity capacitor in a defibrillator is charged to a high voltage and then rapidly discharged through the heart tissue. This quick discharge can reset the heart’s electrical state, allowing the natural pacemaker cells to restore normal rhythm.
- Energy Storage and Release: Capacitors are ideal for this application because they can store significant amounts of energy and release it very quickly, which is crucial for effective defibrillation.
Pacemakers
Capacitors are also integral components of pacemakers, which are devices used to regulate the heartbeat.
- Timing Circuits: Capacitors in pacemakers work with timing circuits to ensure electrical impulses are sent to the heart at regular intervals, stimulating the heart to beat at a normal rate.
- Energy Conservation: The capacitors help manage the energy used by the pacemaker, ensuring that the battery lasts as long as possible by regulating the power distribution efficiently.
Electroencephalography (EEG)
In EEG systems, capacitors are used extensively in the filtering circuits that process the electrical signals recorded from the brain.
- Signal Integrity: Capacitors help filter out noise and stabilize the signals obtained from EEG sensors, which are critical for accurate brain activity analysis.
- Coupling and Decoupling: They are used to couple the electrical signals from the scalp to the recording equipment while blocking DC components, ensuring that only the relevant AC frequencies are analyzed.
Medical Imaging
Capacitors are utilized in various medical imaging systems, including X-ray machines and MRI (Magnetic Resonance Imaging).
- High-voltage Applications: In X-ray machines, capacitors store the high voltage required to generate X-rays efficiently.
- Gradient Systems in MRI: In MRI machines, capacitors are part of the gradient systems where they help in the rapid switching of currents, crucial for generating precise magnetic fields necessary for imaging.
Tissue Engineering anDielectric Materialsd Electroporation
Capacitors can be used in biomedical research areas such as tissue engineering and electroporation.
- Electroporation: This technique involves applying a high-intensity electric field to cells to increase the permeability of the cell membrane, facilitated by capacitors. It is used for introducing substances like drugs or DNA into cells.
- Controlled Energy Delivery: Capacitors provide the precise control over the timing and intensity of the electric field applied during electroporation, crucial for maintaining cell viability while achieving effective permeabilization.
Lab-on-a-chip Systems
In miniaturized devices designed for medical diagnostics, known as lab-on-a-chip systems, capacitors can be employed for various functions:
- Fluid Manipulation: Capacitors can be used to manipulate fluids at the microscale using electrokinetic phenomena.
- Signal Processing: They are crucial for processing electrical signals generated by the sensor components of the chip.
Key Properties of Capacitors: Capacitance and Dielectric Materials
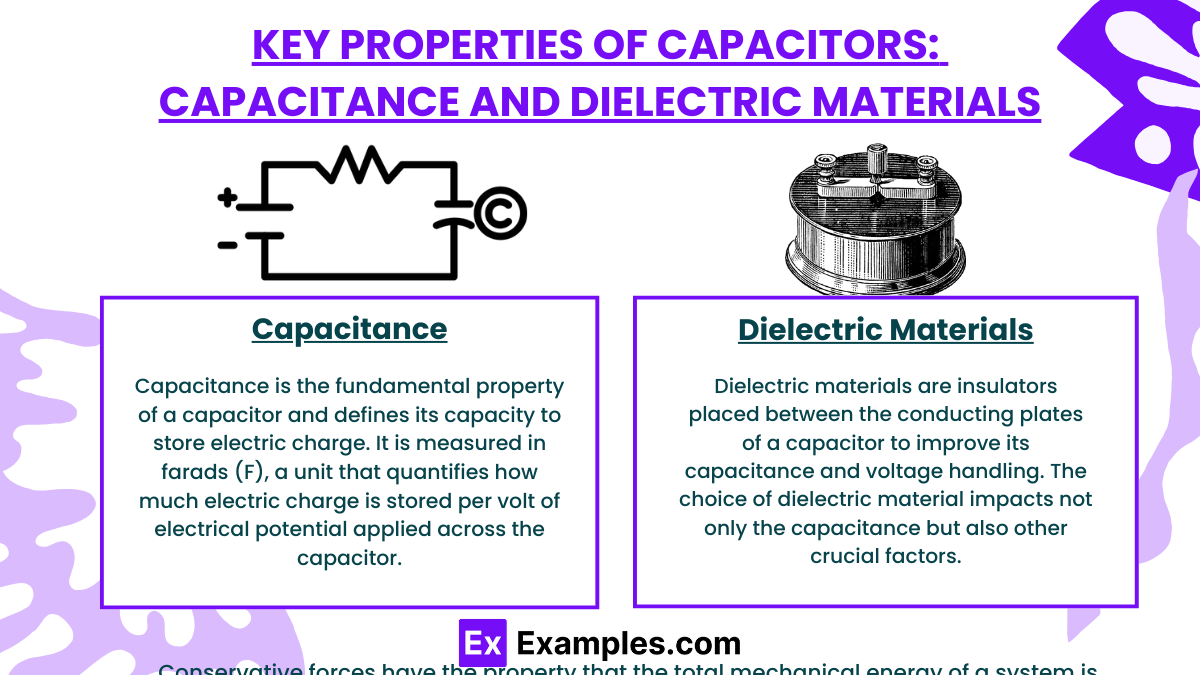
Capacitance
Capacitance is the fundamental property of a capacitor and defines its capacity to store electric charge. It is measured in farads (F), a unit that quantifies how much electric charge is stored per volt of electrical potential applied across the capacitor.
Determining Factors:
- Area of the Plates (A): The capacitance is directly proportional to the area of the conducting plates. Larger plate areas provide more space for storing charge, thus increasing capacitance.
- Distance Between the Plates (d): Capacitance is inversely proportional to the distance between the plates. Closer plates result in a stronger electric field for a given charge, increasing capacitance.
- Dielectric Constant (κ): The material between the plates, known as the dielectric, also affects capacitance. The dielectric constant of the material is a measure of its ability to increase the capacitance compared to empty space (vacuum).
The capacitance of a parallel-plate capacitor can be calculated with the formula: C = κϵ0A/d where:
- ϵ0 is the permittivity of free space (approximately 8.85×10−12,
- κ is the relative permittivity or dielectric constant of the material between the plates,
- A is the area of one of the plates,
- d is the separation between the two plates.
Dielectric Materials
Dielectric materials are insulators placed between the conducting plates of a capacitor to improve its capacitance and voltage handling. The choice of dielectric material impacts not only the capacitance but also other crucial factors such as the capacitor’s size, temperature stability, and frequency response.
Key Properties of Dielectric Materials:
- Dielectric Constant (Relative Permittivity): A higher dielectric constant means the material can store more charge at a given voltage, effectively increasing the capacitance.
- Dielectric Strength: This is the maximum electric field a dielectric material can withstand without breaking down. Higher dielectric strength allows a capacitor to operate at higher voltages.
- Loss Tangent (Dissipation Factor): This property measures how much energy from the electric field is lost as heat within the dielectric. Lower values indicate more efficient capacitors with minimal energy losses.
Common Dielectric Materials:
- Ceramic: Used in ceramic capacitors, offering high frequency characteristics and good temperature stability. They are commonly used in high-frequency applications like RF circuitry.
- Polyester and Polypropylene: These plastics are used in film capacitors and are valued for their stability and low cost. They are commonly used in power systems and audio electronics.
- Tantalum: Tantalum capacitors have high capacitance values and are very stable, making them ideal for filtering applications in power supply circuits in compact electronic devices.
- Electrolytic: Aluminum and tantalum electrolytic capacitors have a very high capacitance-to-volume ratio, suited for applications requiring large capacitance values, such as in power smoothing circuits.
Examples
Example 1: Power Supply Smoothing
Capacitors are commonly used in power supply circuits to smooth out voltage fluctuations. When alternating current (AC) is converted to direct current (DC), capacitors store excess charge and release it when the voltage dips, ensuring a more stable output. This smoothing action helps prevent voltage spikes and provides a consistent power supply for electronic devices.
Example 2: Timing Circuits
In timing applications, capacitors work in conjunction with resistors to create delays. For example, in a simple RC (resistor-capacitor) timing circuit, a capacitor charges and discharges at a rate determined by the resistance and capacitance values. This principle is used in various applications, such as generating time delays in blinking LED circuits or timing events in digital devices.
Example 3: Filters in Audio Equipment
Capacitors are essential components in audio equipment, where they serve as filters. In crossover networks for speakers, capacitors can block certain frequencies while allowing others to pass, ensuring that high-frequency signals reach tweeters and low-frequency signals reach woofers. This filtering enhances sound quality and protects speakers from damage due to inappropriate frequency ranges.
Example 4: Energy Storage in Flash Cameras
Capacitors are used in camera flash systems to store energy and release it quickly for a brief, intense burst of light. When the flash is triggered, the stored energy in the capacitor discharges rapidly through the flash bulb, providing the necessary brightness for capturing well-lit images in low-light conditions. This rapid discharge highlights the capacitor’s ability to store and release energy efficiently.
Example 5: Motor Start Capacitors
In electric motors, especially single-phase motors, capacitors help start the motor by providing an initial boost of energy. The motor start capacitor stores energy and releases it to create a phase shift in the current, allowing the motor to start smoothly and efficiently. Once the motor reaches a certain speed, the capacitor is typically disconnected from the circuit, demonstrating its role in enhancing motor performance.
Practice Questions
Question 1
What is the primary function of a capacitor in an electrical circuit?
A) To amplify current
B) To store and release electrical energy
C) To convert AC to DC
D) To measure voltage
Correct Answer: B) To store and release electrical energy.
Explanation: The primary function of a capacitor is to store electrical energy in an electric field when a voltage is applied across its plates. This stored energy can then be released back into the circuit when needed. Capacitors do not amplify current, convert AC to DC, or measure voltage; they simply store energy for various applications in circuits.
Question 2
In which of the following applications would you typically find a timing capacitor?
A) In a battery charger
B) In a flash camera
C) In a filter circuit for audio equipment
D) In a delay circuit for LED blinking
Correct Answer: D) In a delay circuit for LED blinking.
Explanation: Timing capacitors are often used in circuits designed to create delays, such as those that cause an LED to blink. In an RC timing circuit, the capacitor charges and discharges at a rate determined by its capacitance and the resistance in the circuit, creating a time delay. While capacitors are also used in battery chargers, flash cameras, and audio filters, the context of creating a delay specifically highlights their function in timing applications.
Question 3
What effect does connecting capacitors in parallel have on the total capacitance of the circuit?
A) It decreases the total capacitance.
B) It does not change the total capacitance.
C) It increases the total capacitance.
D) It creates a short circuit.
Correct Answer: C) It increases the total capacitance.
Explanation: When capacitors are connected in parallel, the total capacitance increases because each capacitor adds its capacitance to the total. In parallel connections, the voltage across each capacitor remains the same, but the effective area for storing charge increases, leading to a higher overall capacitance. This principle is fundamental in designing circuits that require specific capacitance values. The other options are incorrect; the total capacitance does not decrease or remain constant in parallel, nor does it create a short circuit.