Preparing for the MCAT requires a thorough understanding of the cell membrane, a key concept within the Cells foundation. Mastery of membrane structure, transport mechanisms, and fluidity is essential. This knowledge provides insights into cellular communication, homeostasis, and nutrient exchange, critical for achieving a high MCAT score and excelling in biological sciences.
Learning Objective
In studying “Cell Membrane Overview” for the MCAT, you should learn to understand the fundamental structure and components of cell membranes, including the phospholipid bilayer, embedded proteins, and cholesterol. Analyze how the membrane regulates transport through passive diffusion, facilitated diffusion, and active transport. Evaluate key membrane properties such as fluidity, selective permeability, and signal transduction. Additionally, explore how integral proteins, ion channels, and receptors mediate communication between cells and their environment. Apply this knowledge to understanding homeostasis, membrane potential, and osmoregulation, and use your understanding to interpret experimental data in MCAT practice questions related to cellular physiology and function.
Structure and Components of the Cell Membrane
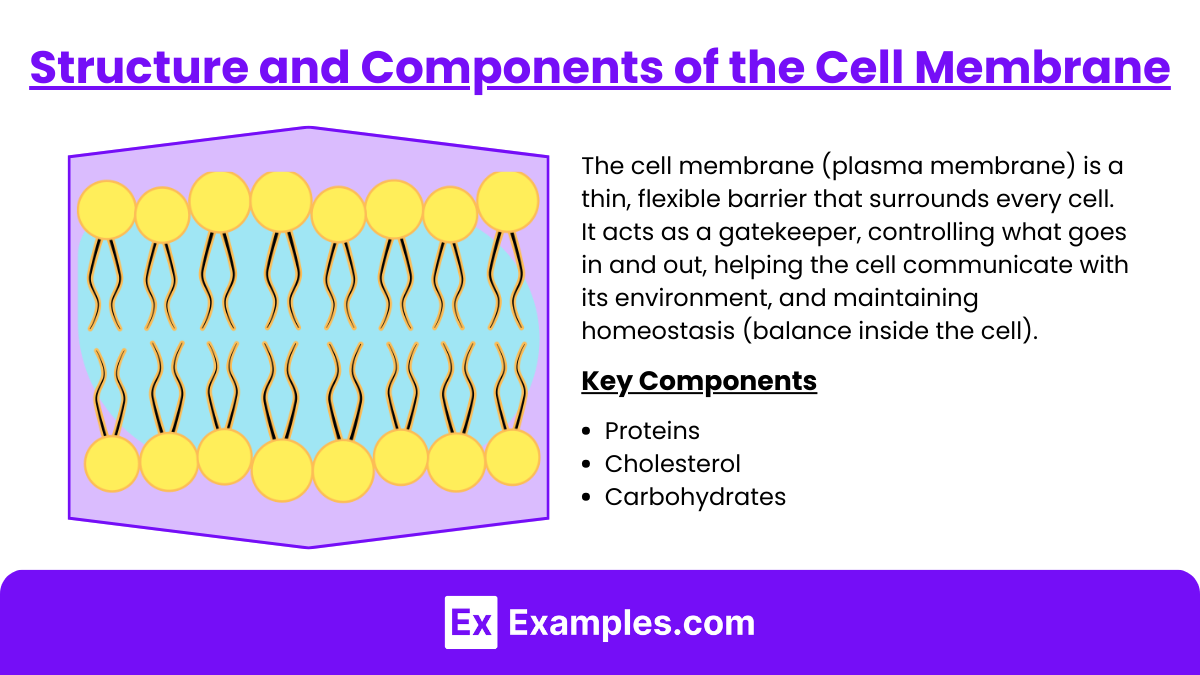
The cell membrane (plasma membrane) is a thin, flexible barrier that surrounds every cell. It acts as a gatekeeper, controlling what goes in and out, helping the cell communicate with its environment, and maintaining homeostasis (balance inside the cell).
Structure of the Cell Membrane:
- Phospholipid Bilayer
The core of the membrane is made of phospholipids arranged in a bilayer:- Heads: Hydrophilic (water-attracting) – face outward, towards water.
- Tails: Hydrophobic (water-repelling) – point inward, away from water.
Key Components of the Cell Membrane:
- Proteins
- Integral (Transmembrane) Proteins: Span the membrane from one side to the other.
Function: Act as channels, transporters, or receptors. - Peripheral Proteins: Sit on the surface of the membrane.
Function: Help with signaling or maintaining the cell’s shape.
- Integral (Transmembrane) Proteins: Span the membrane from one side to the other.
- Cholesterol
Found between phospholipids.
Function:- Makes the membrane stronger and more stable.
- Prevents it from becoming too fluid or too rigid with temperature changes.
- Carbohydrates
Attached to proteins (forming glycoproteins) or lipids (forming glycolipids).
Function:- Help with cell recognition (like an ID card for the cell).
- Play a role in immune responses and cell-to-cell communication.
Transport Mechanisms across the Cell Membrane
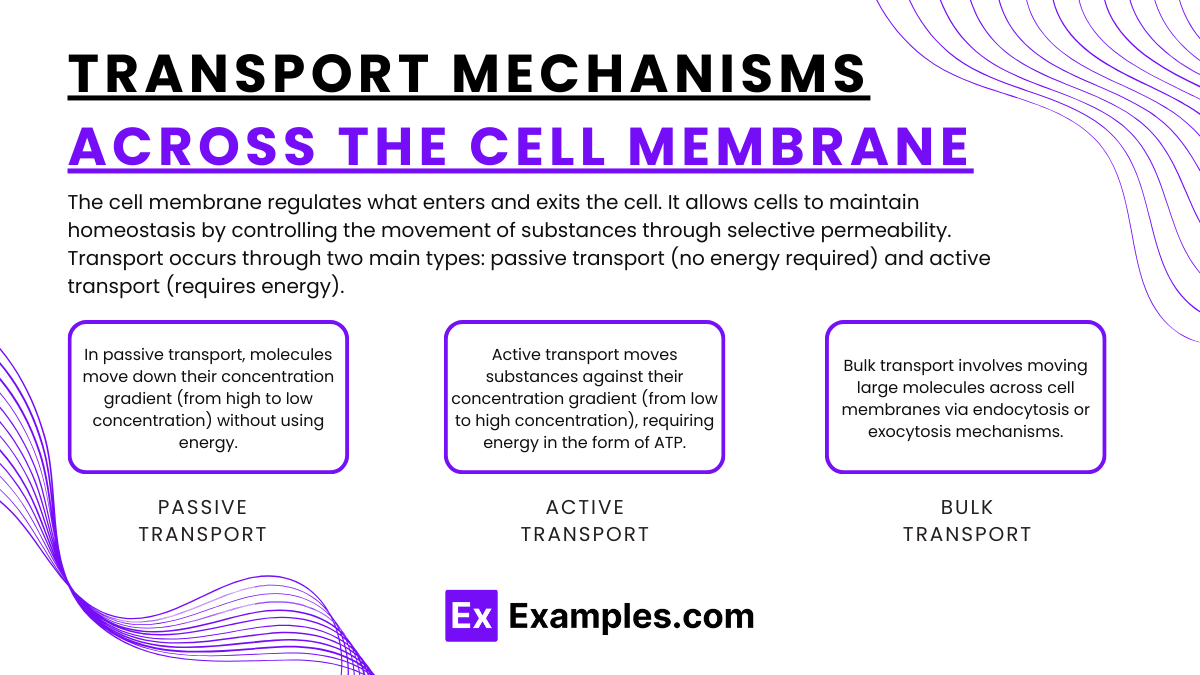
The cell membrane regulates what enters and exits the cell. It allows cells to maintain homeostasis by controlling the movement of substances through selective permeability. Transport occurs through two main types: passive transport (no energy required) and active transport (requires energy).
1. Passive Transport (No Energy Needed)
In passive transport, molecules move down their concentration gradient (from high to low concentration) without using energy.
a) Simple Diffusion
- What happens? Small, non-polar molecules pass directly through the phospholipid bilayer.
- Examples: Oxygen (O₂), Carbon dioxide (CO₂).
- Key Idea: No proteins or energy are required.
b) Facilitated Diffusion
- What happens? Larger or polar molecules move through channel or carrier proteins.
- Examples: Glucose, ions (like Na⁺, K⁺).
- Key Idea: Requires transport proteins but no energy.
c) Osmosis
- What happens? Water molecules move through a semipermeable membrane from low solute concentration to high solute concentration.
- Example: Water moving into a dehydrated cell.
- Key Idea: Uses special aquaporin proteins for faster water transport.
2. Active Transport (Requires Energy – ATP)
Active transport moves substances against their concentration gradient (from low to high concentration), requiring energy in the form of ATP.
a) Primary Active Transport
- What happens? ATP is directly used to pump molecules across the membrane.
- Example: Sodium-potassium pump (Na⁺/K⁺ pump) moves Na⁺ out of the cell and K⁺ in.
- Key Idea: Maintains ion gradients crucial for nerve function and muscle contraction.
b) Secondary Active Transport (Co-transport)
- What happens? One molecule moves down its gradient to power the movement of another molecule against its gradient.
- Example: Glucose-Na⁺ symport, where Na⁺ moves down its gradient, pulling glucose into the cell with it.
- Key Idea: Uses energy indirectly from the primary gradient.
3. Bulk Transport (Large Molecules)
Bulk transport involves moving large molecules across cell membranes via endocytosis or exocytosis mechanisms.
a) Endocytosis
- What happens? The membrane engulfs substances and forms a vesicle inside the cell.
- Types:
- Phagocytosis: “Cell eating” (e.g., engulfing bacteria).
- Pinocytosis: “Cell drinking” (e.g., taking in fluids).
- Example: White blood cells engulfing pathogens.
b) Exocytosis
- What happens? Vesicles fuse with the membrane and release contents outside the cell.
- Example: Release of neurotransmitters from nerve cells.
- Key Idea: Helps with waste removal and sending signals to other cells.
Membrane Properties: Fluidity, Permeability, and Selectivity
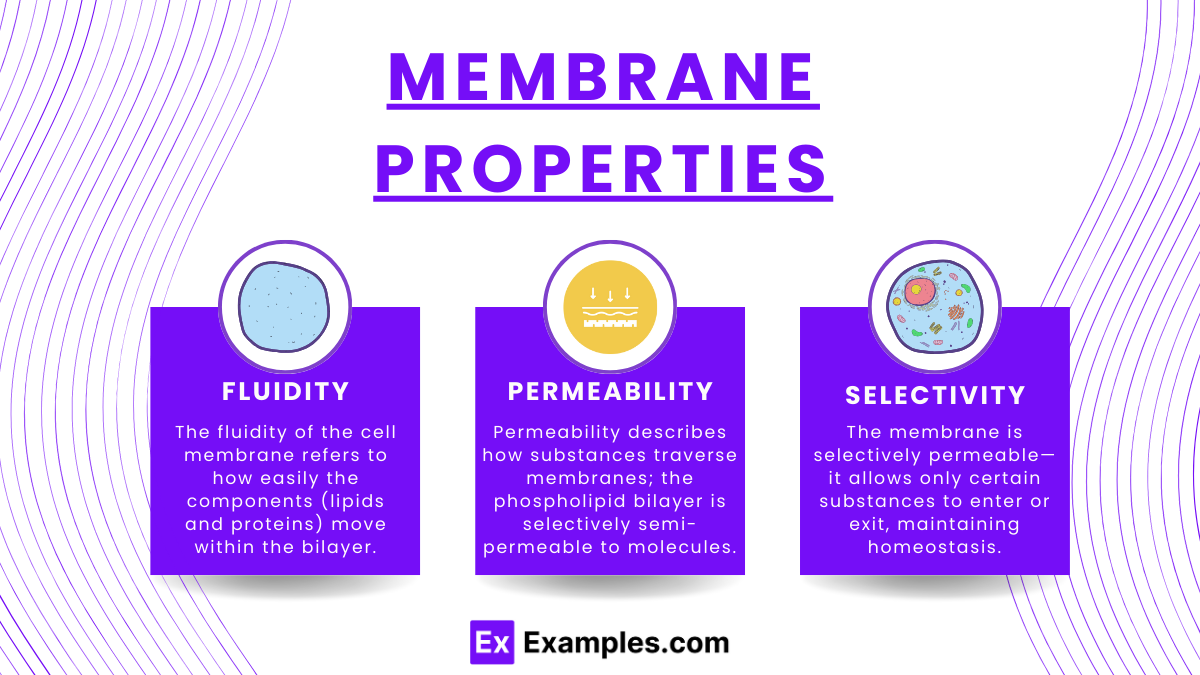
1. Fluidity
- The fluidity of the cell membrane refers to how easily the components (lipids and proteins) move within the bilayer.
- Increases:
- High temperature
- Unsaturated fatty acids (kinks prevent tight packing)
- Decreases:
- Low temperature
- Saturated fatty acids (tightly packed)
- Cholesterol (reduces fluidity at high temps; maintains fluidity at low temps)
- Importance: Allows membrane repair, protein movement, and temperature adaptation.
2. Permeability
- Permeability refers to how easily substances can pass through the membrane. The phospholipid bilayer allows some molecules to pass more freely than others, making the membrane semi-permeable.
- Can Pass Freely: Small, non-polar molecules (O₂, CO₂), lipid-soluble molecules (steroids).
- Cannot Pass Freely: Large polar molecules (glucose), ions (Na⁺, K⁺).
- Importance: Controls the movement of nutrients, gases, and ions.
3. Selectivity
- The membrane is selectively permeable—it allows only certain substances to enter or exit, maintaining homeostasis.
- Factors:
- Transport Proteins: Channels (for ions) and carriers (for glucose).
- Receptor Proteins: Detect signals (e.g., hormones).
- Lipid Composition: Adjusts for environmental needs.
- Importance: Maintains homeostasis and enables proper cell responses to signals.
Clinical and Experimental Applications
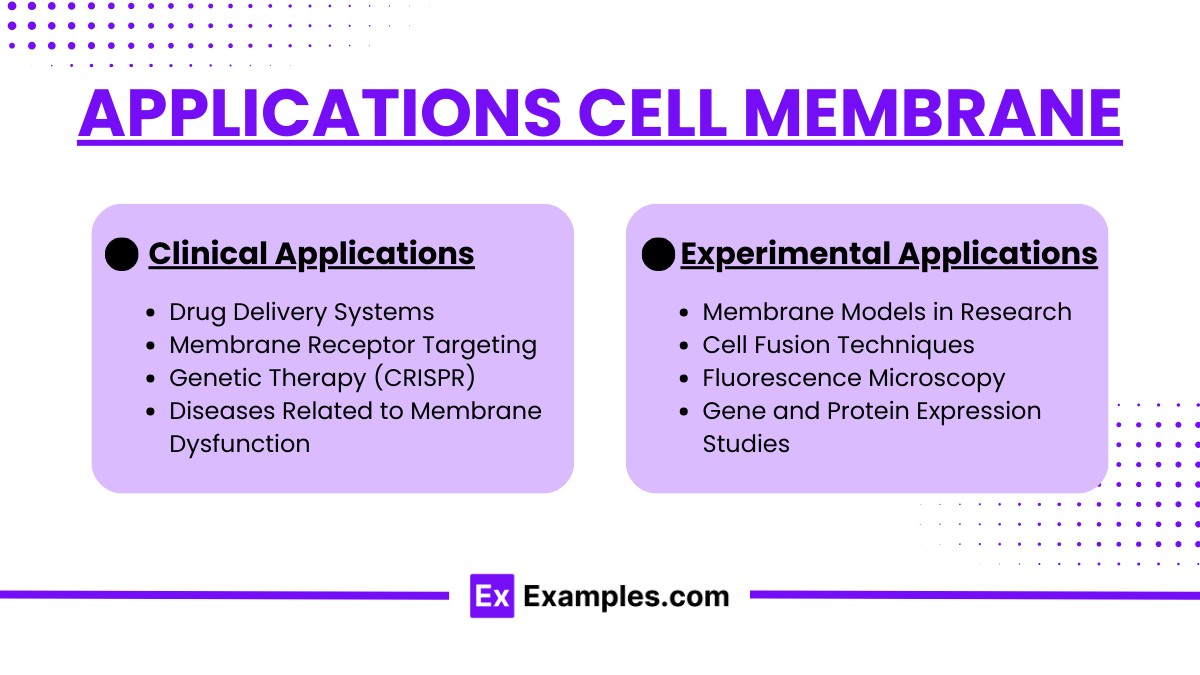
Clinical Applications
- Drug Delivery Systems
- Lipid-based nanoparticles (e.g., liposomes) are used to deliver drugs by fusing with cell membranes.
- Membrane permeability determines how well drugs are absorbed (e.g., oral medications need to pass through intestinal membranes).
- Membrane Receptor Targeting
- Receptor proteins on membranes are targeted by drugs (e.g., insulin binds to insulin receptors to control glucose uptake).
- Antibody therapies (e.g., monoclonal antibodies) target cancer cell membranes.
- Genetic Therapy (CRISPR)
- Lipid vesicles and viral vectors help deliver CRISPR components into cells to modify genes.
- Diseases Related to Membrane Dysfunction
- Cystic fibrosis: Caused by defective chloride ion channels (CFTR protein).
- Hypercholesterolemia: Results from faulty cholesterol receptors, leading to excess cholesterol in the blood.
Experimental Applications
- Membrane Models in Research
- Artificial membranes (lipid bilayers) are used to study how drugs and ions cross membranes.
- Patch-clamp technique measures ion flow through channels, critical for understanding nerve impulses.
- Cell Fusion Techniques
- Used in the production of monoclonal antibodies by fusing immune cells with cancer cells (hybridomas).
- Fluorescence Microscopy
- Membrane-bound dyes help visualize how proteins and lipids move within the membrane (fluidity studies).
- Gene and Protein Expression Studies
- Electroporation: Electrical pulses create temporary pores in membranes to introduce DNA or drugs into cells.
Examples
Example 1: Sodium-Potassium Pump (Na+/K+ Pump)
- Description: This pump is an example of active transport that moves 3 Na⁺ ions out of the cell and 2 K⁺ ions into the cell, maintaining the resting membrane potential.
- Application: Helps regulate nerve impulses and muscle contractions by creating electrochemical gradients.
Example 2: Osmosis in Red Blood Cells
- Description: When red blood cells are placed in a hypertonic solution (high solute concentration), water moves out, causing the cells to shrink. In a hypotonic solution (low solute concentration), water enters, causing the cells to swell and burst.
- Application: Demonstrates how cell membranes regulate water balance through selective permeability.
Example 3: Facilitated Diffusion of Glucose
- Description: Glucose cannot cross the cell membrane directly because it is polar, so it uses GLUT transport proteins for facilitated diffusion.
- Application: Important in tissues like muscle and liver, ensuring glucose uptake for cellular respiration.
Example 4: G-Protein Coupled Receptor (GPCR) Signaling
- Description: A hormone (e.g., adrenaline) binds to a membrane receptor, activating a G-protein, which then triggers downstream signaling pathways inside the cell.
- Application: Explains how cells respond to external stimuli and regulate processes like heart rate and energy metabolism.
Example 5: Cystic Fibrosis and Defective Ion Channels
- Description: Cystic fibrosis results from a mutation in the CFTR gene, causing chloride ion channels to malfunction. This leads to thick mucus accumulation in organs like the lungs.
- Application: Highlights the importance of membrane proteins in ion transport and how defects can result in disease.
Practice Questions:
Question 1:
Which of the following processes is an example of passive transport across the cell membrane?
A) Sodium-potassium pump
B) Exocytosis
C) Facilitated diffusion
D) Endocytosis
Answer: C) Facilitated diffusion
Explanation: Passive transport occurs without the use of cellular energy. Facilitated diffusion allows molecules, like glucose or ions, to cross the membrane through specific channel or carrier proteins along the concentration gradient. The sodium-potassium pump, exocytosis, and endocytosis require energy, classifying them as active transport processes.
Question 2:
Which type of membrane transport uses energy directly from ATP?
A) Osmosis
B) Simple diffusion
C) Sodium-potassium pump
D) Facilitated diffusion
Answer: C) Sodium-potassium pump
Explanation: The sodium-potassium pump is an example of primary active transport. It moves sodium and potassium ions against their concentration gradients by hydrolyzing ATP. In contrast, osmosis, simple diffusion, and facilitated diffusion do not require energy and occur passively along concentration gradients.
Question 3:
Which membrane component is primarily responsible for the selective permeability of the plasma membrane?
A) Phospholipid bilayer
B) Cholesterol molecules
C) Glycoproteins
D) Integral proteins
Answer: A) Phospholipid bilayer
Explanation: The phospholipid bilayer forms the structural foundation of the cell membrane, allowing selective permeability. The hydrophobic tails restrict the passage of polar molecules, while small non-polar molecules can diffuse freely. Integral proteins assist in transporting specific molecules, but it’s the bilayer that creates the membrane’s primary barrier function.