Enzymes are biological catalysts that accelerate chemical reactions by lowering activation energy, making them essential for sustaining life. They exhibit high specificity for substrates and operate through mechanisms such as the lock-and-key or induced fit models. Enzymes play vital roles in metabolism, digestion, DNA replication, and energy production. Factors like temperature, pH, and inhibitors influence their activity.
Learning Objectives
In studying “Enzymes” for the MCAT, you should learn to understand enzyme structure, function, and kinetics, including concepts like the Michaelis-Menten equation and enzyme inhibition. Analyze how enzymes lower activation energy, catalyze biological reactions, and exhibit specificity through models like the lock-and-key and induced fit. Evaluate regulatory mechanisms such as allosteric modulation, feedback inhibition, and covalent modification. Additionally, explore the clinical significance of enzymes in diagnostics and therapeutics, such as enzyme deficiencies and drug targets. Apply this understanding to interpret enzyme behavior, kinetic data, and inhibition patterns in experimental setups and MCAT-style passages.
Overview of Enzymes
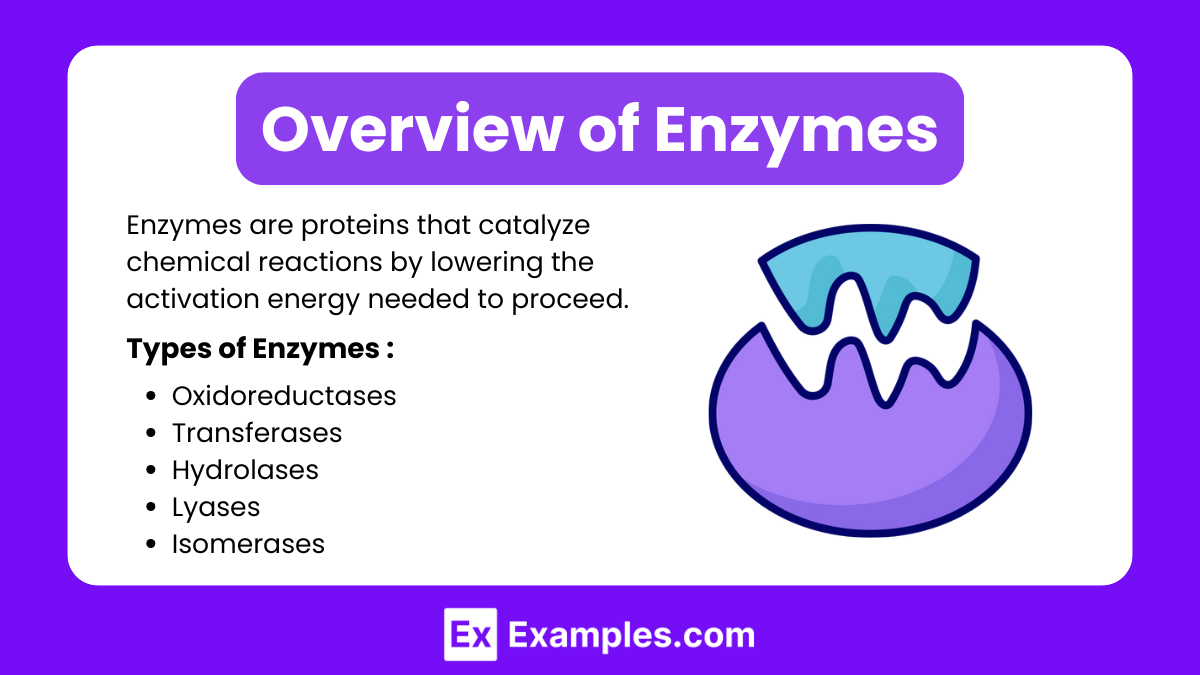
Enzymes are biological macromolecules, typically proteins (though some RNA molecules act as enzymes, known as ribozymes), that catalyze chemical reactions by lowering the activation energy required for the reaction to proceed. They are essential in various physiological processes, such as digestion, energy production, DNA replication, and cell signaling. Without enzymes, most biochemical reactions would occur too slowly to sustain life.
Types of Enzymes (Based on Function)
- Oxidoreductases: Facilitate oxidation-reduction (redox) reactions (e.g., dehydrogenases).
- Transferases: Transfer functional groups between molecules (e.g., kinases).
- Hydrolases: Catalyze hydrolysis reactions, breaking bonds with water (e.g., lipase, protease).
- Lyases: Break bonds without hydrolysis or oxidation (e.g., decarboxylases).
- Isomerases: Rearrange atoms within a molecule (e.g., phosphoglucoisomerase).
Structure and Function of Enzymes
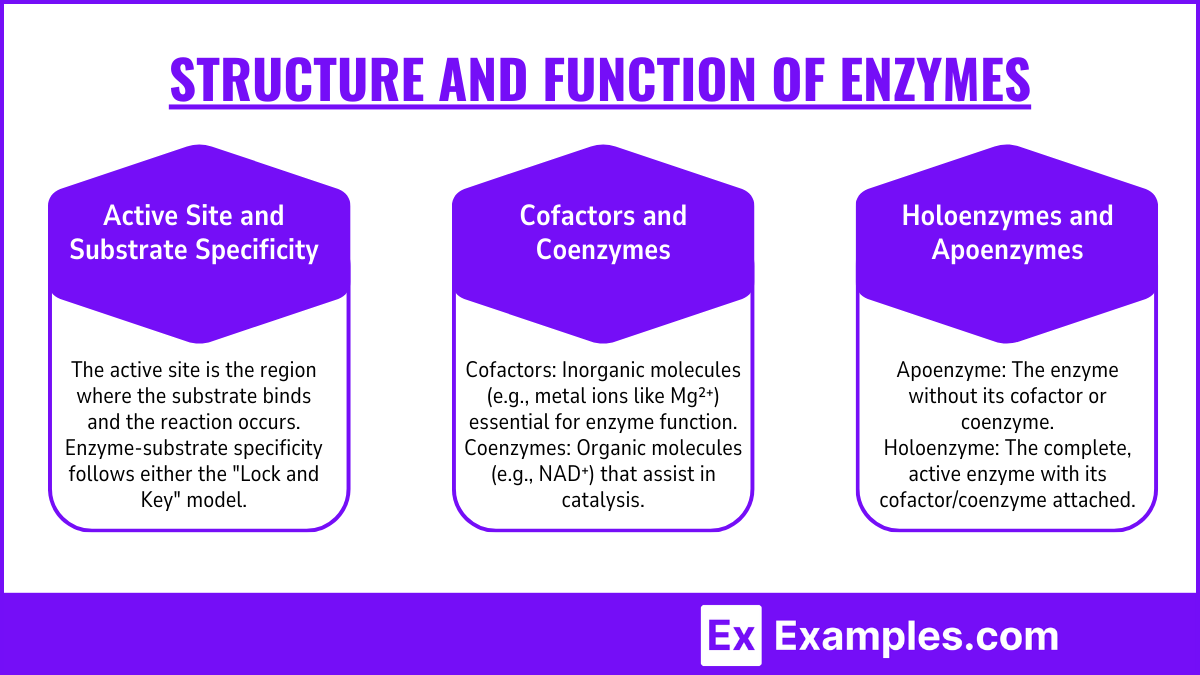
- Active Site and Substrate Specificity:
- The active site is the region where the substrate binds and the reaction occurs.
- Enzyme-substrate specificity follows either the “Lock and Key” model or the “Induced Fit” model:
- Lock and Key: Substrate fits exactly into the active site.
- Induced Fit: Enzyme adjusts its shape to fit the substrate upon binding.
- Cofactors and Coenzymes:
- Cofactors: Inorganic molecules (e.g., metal ions like Mg²⁺) essential for enzyme function.
- Coenzymes: Organic molecules (e.g., NAD⁺, FAD) that assist in catalysis by transferring groups between reactions.
- Prosthetic Groups: Tightly bound cofactors that remain attached to the enzyme.
- Holoenzymes and Apoenzymes:
- Apoenzyme: The enzyme without its cofactor or coenzyme.
- Holoenzyme: The complete, active enzyme with its cofactor/coenzyme attached.
Mechanism of Enzyme Action
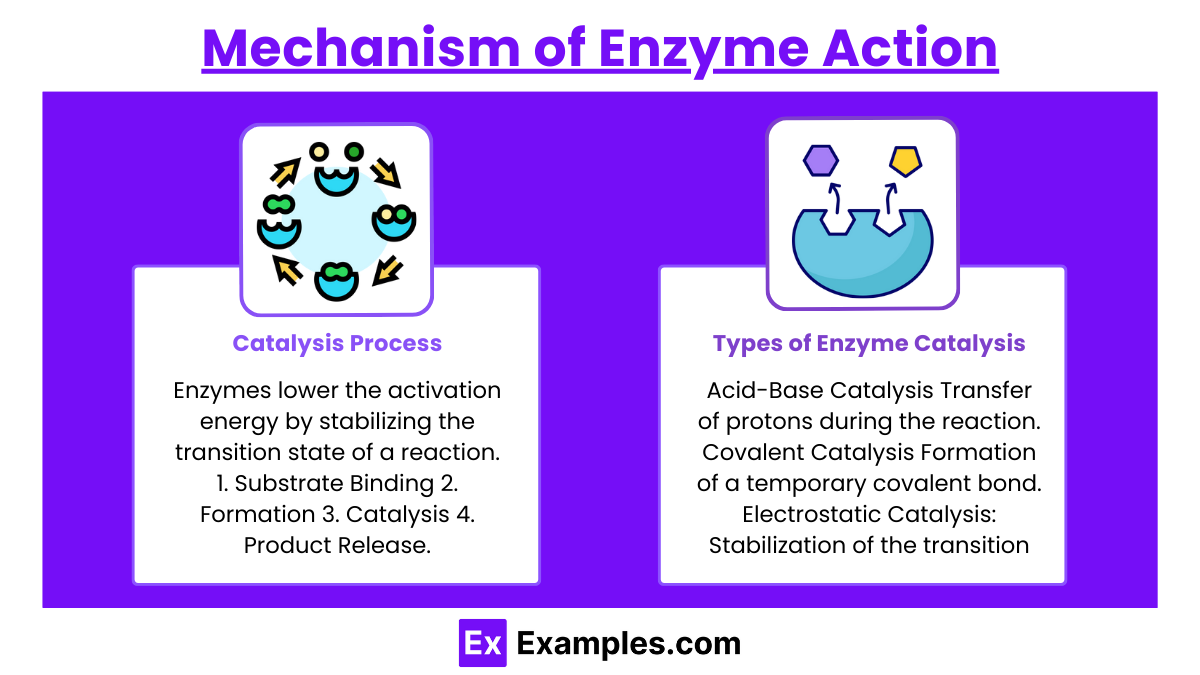
- Catalysis Process:
- Enzymes lower the activation energy by stabilizing the transition state of a reaction.
- Steps:
- Substrate Binding: Substrate binds to the enzyme’s active site.
- Formation of the Enzyme-Substrate Complex: Enzyme changes shape slightly (induced fit).
- Catalysis: Substrate is converted into the product.
- Product Release: Products leave the enzyme, making it available for new reactions.
- Types of Enzyme Catalysis:
- Acid-Base Catalysis: Transfer of protons during the reaction.
- Covalent Catalysis: Formation of a temporary covalent bond between the enzyme and substrate.
- Electrostatic Catalysis: Stabilization of the transition state by ionic interactions.
Factors Affecting Enzyme Activity
- Temperature:
- Enzymes have an optimal temperature where activity is highest. Too high a temperature leads to denaturation.
- pH:
- Each enzyme has an optimal pH range. Outside this range, the enzyme structure may change, affecting function.
- Substrate Concentration:
- Enzyme activity increases with substrate concentration until the enzyme becomes saturated.
- Inhibitors:
- Competitive Inhibition: Inhibitor competes with the substrate for the active site.
- Non-Competitive Inhibition: Inhibitor binds to a different site, altering the enzyme’s function.
- Uncompetitive Inhibition: Inhibitor binds only to the enzyme-substrate complex.
Regulation of Enzymes
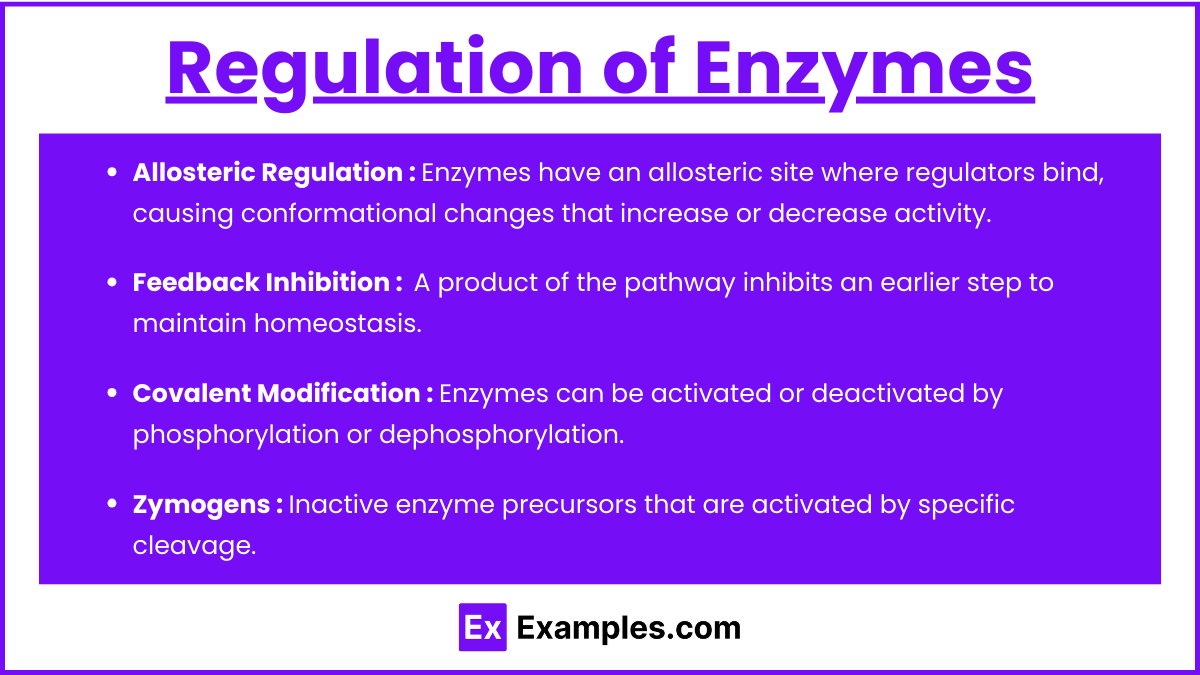
- Allosteric Regulation:
- Enzymes have an allosteric site where regulators bind, causing conformational changes that increase or decrease activity.
- Feedback Inhibition:
- A product of the pathway inhibits an earlier step to maintain homeostasis.
- Covalent Modification:
- Enzymes can be activated or deactivated by phosphorylation or dephosphorylation.
- Zymogens:
- Inactive enzyme precursors that are activated by specific cleavage.
Examples
Example 1 : Amylase
Amylase is a digestive enzyme that breaks down starch and glycogen into simpler sugars like maltose and glucose. It is secreted by both the salivary glands (salivary amylase) and the pancreas (pancreatic amylase) to aid in carbohydrate digestion. The MCAT often emphasizes its function in the context of metabolism and digestion.
Example 2 : Pepsin
Pepsin is a protease enzyme responsible for breaking down proteins into peptides in the acidic environment of the stomach. It is secreted as the inactive zymogen pepsinogen, which is activated by stomach acid (HCl). Understanding zymogen activation and enzyme specificity is crucial for MCAT preparation.
Example 3 : DNA Polymerase
DNA polymerase is a key enzyme involved in DNA replication, responsible for synthesizing new DNA strands by adding nucleotides to the growing chain. It plays a crucial role during the S-phase of the cell cycle, ensuring accurate replication of genetic material. The MCAT covers DNA polymerase in contexts related to genetics, molecular biology, and mutations.
Example 4 : Lactase
Lactase is an enzyme found in the small intestine, where it hydrolyzes lactose, the sugar in milk, into glucose and galactose. Lactose intolerance, caused by a deficiency of lactase, is often discussed on the MCAT in relation to enzyme deficiency disorders and metabolism.
Example 5 : ATP Synthase
ATP synthase is an essential enzyme located in the mitochondrial inner membrane, where it catalyzes the formation of ATP from ADP and inorganic phosphate during oxidative phosphorylation. It plays a critical role in cellular respiration and energy production, making it a frequently tested enzyme on the MCAT.
Practice Questions
Question 1
Which of the following models best explains how enzymes interact with their substrates?
A) Lock and Key Model
B) Induced Fit Model
C) Fluid Mosaic Model
D) Allosteric Model
Correct Answer: B) Induced Fit Model
Explanation: The Induced Fit Model states that an enzyme’s active site undergoes a conformational change upon substrate binding, allowing a more precise fit. This flexibility enhances the enzyme’s ability to catalyze the reaction. The Lock and Key Model (option A) assumes that the substrate fits exactly without any change in shape, which is a simplified explanation. The Fluid Mosaic Model (option C) describes cell membrane structure, making it irrelevant here. The Allosteric Model (option D) explains regulation through binding at sites other than the active site, not enzyme-substrate interaction.
Question 2
Which of the following statements is correct about the Michaelis-Menten constant (Kₘ)?
A) It is the substrate concentration at which the enzyme is fully saturated.
B) It reflects the affinity of the enzyme for its substrate.
C) A lower Kₘ indicates a lower affinity for the substrate.
D) Kₘ is independent of the substrate concentration.
Correct Answer: B) It reflects the affinity of the enzyme for its substrate.
Explanation: Kₘ represents the substrate concentration at which the reaction rate is half of Vₘₐₓ. It serves as an indicator of enzyme-substrate affinity: a lower Kₘ suggests higher affinity, as less substrate is needed to reach half the maximum velocity. Option A is incorrect because enzymes can still process substrates beyond this concentration. Option C is false, as a lower Kₘ indicates higher affinity. Option D is misleading; while Kₘ depends on enzyme-substrate interactions, it is often treated as independent of substrate concentration in a specific reaction.
Question 3
What type of inhibition occurs when an inhibitor binds only to the enzyme-substrate complex, reducing both the Vₘₐₓ and Kₘ values?
A) Competitive Inhibition
B) Non-Competitive Inhibition
C) Uncompetitive Inhibition
D) Mixed Inhibition
Correct Answer: C) Uncompetitive Inhibition.
Explanation: In uncompetitive inhibition, the inhibitor binds exclusively to the enzyme-substrate complex, preventing the complex from releasing products. This reduces both Kₘ (since the substrate appears to bind more easily) and Vₘₐₓ (since fewer active enzyme-substrate complexes can complete the reaction). Competitive inhibition (option A) involves the inhibitor competing for the active site, affecting only Kₘ. Non-competitive inhibition (option B) reduces Vₘₐₓ but not Kₘ, as the inhibitor binds to a different site. Mixed inhibition (option D) can alter both parameters but not in the same way as uncompetitive inhibition.