Preparing for the MCAT involves understanding evolution and population dynamics, fundamental topics in biology. This area explores the mechanisms of natural selection, genetic drift, and gene flow, along with the principles governing population growth and interactions. It covers concepts like adaptation, speciation, Hardy-Weinberg equilibrium, and the influence of environmental pressures on allele frequencies. Additionally, it emphasizes the role of evolutionary mechanisms in shaping genetic diversity and species behavior. Mastery of evolution and population dynamics is vital for a high MCAT score, as it relates to species survival, genetic variation, and ecological balance.
Learning Objectives
When studying “Evolution and Population Dynamics” for the MCAT, focus on understanding the mechanisms of evolution (natural selection, genetic drift, gene flow, mutation) and Hardy-Weinberg equilibrium, along with how selection drives adaptation and speciation. Grasp population growth models, carrying capacity, and interactions like competition and symbiosis. Recognize how environmental factors shape evolutionary patterns, emphasizing coevolution and adaptive strategies. This foundational knowledge will help you analyze evolutionary changes and population trends effectively for the MCAT.
1. Introduction to Evolution and Population Dynamics
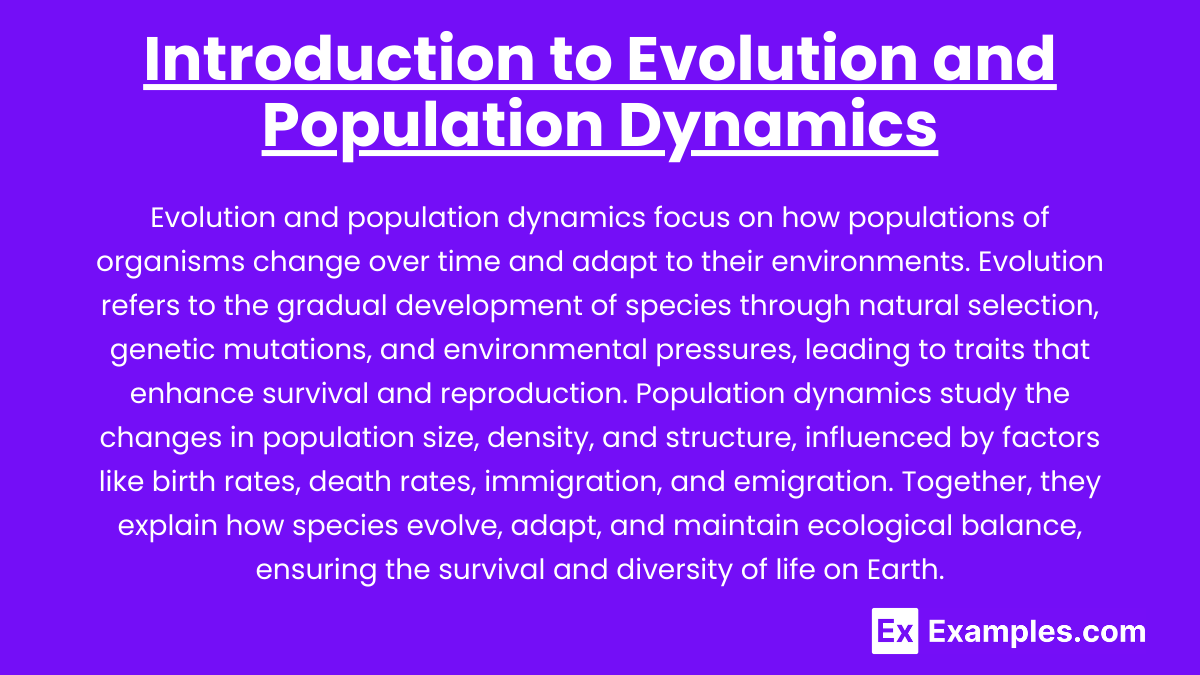
Evolution refers to the process by which species undergo changes over generations due to genetic variation, natural selection, and other mechanisms. Population dynamics studies how populations change over time due to factors like birth rates, death rates, migration, and evolution. In the MCAT, understanding the interplay between evolution and population dynamics helps in analyzing biological and ecological patterns.
2. Core Concepts
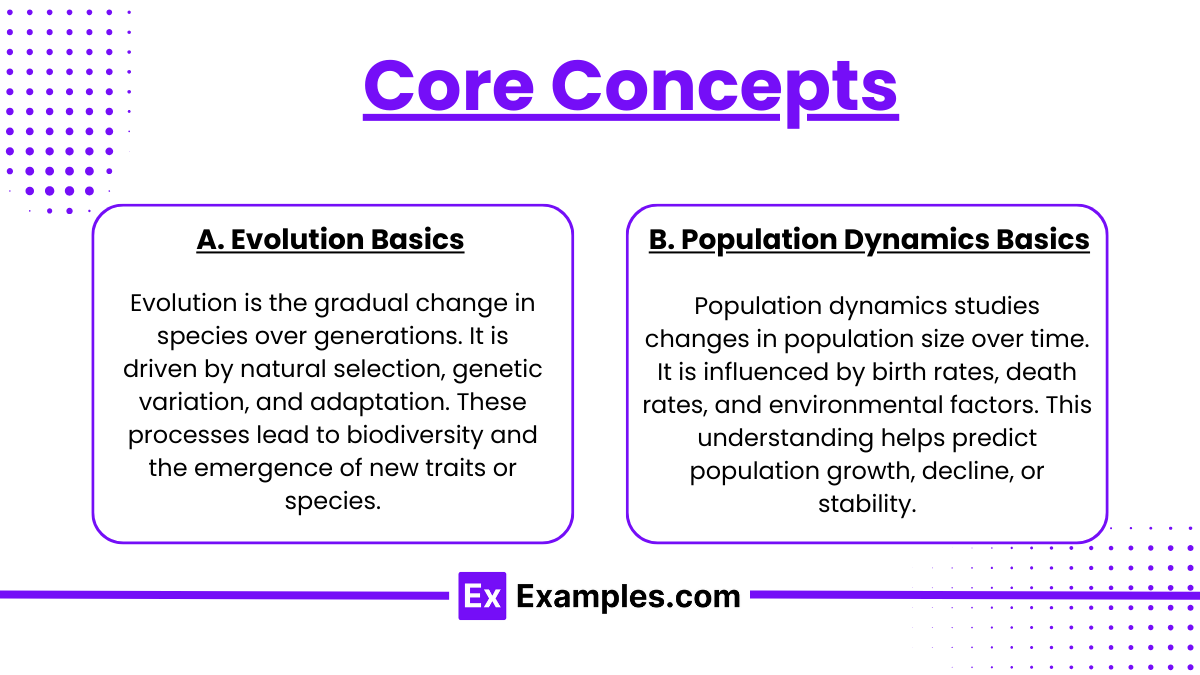
A. Evolution Basics
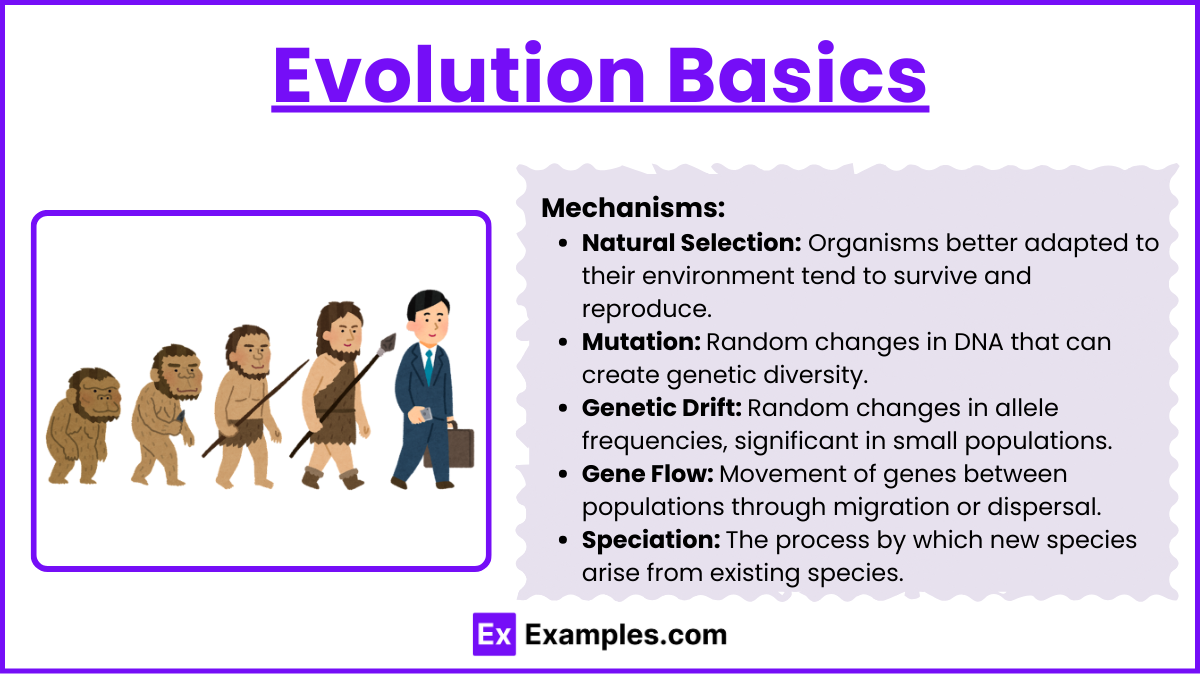
- Definition: Evolution is the change in the heritable traits of biological populations over successive generations.
- Mechanisms:
- Natural Selection: Organisms better adapted to their environment tend to survive and reproduce.
- Mutation: Random changes in DNA that can create genetic diversity.
- Genetic Drift: Random changes in allele frequencies, significant in small populations.
- Gene Flow: Movement of genes between populations through migration or dispersal.
- Speciation: The process by which new species arise from existing species.
B. Population Dynamics Basics
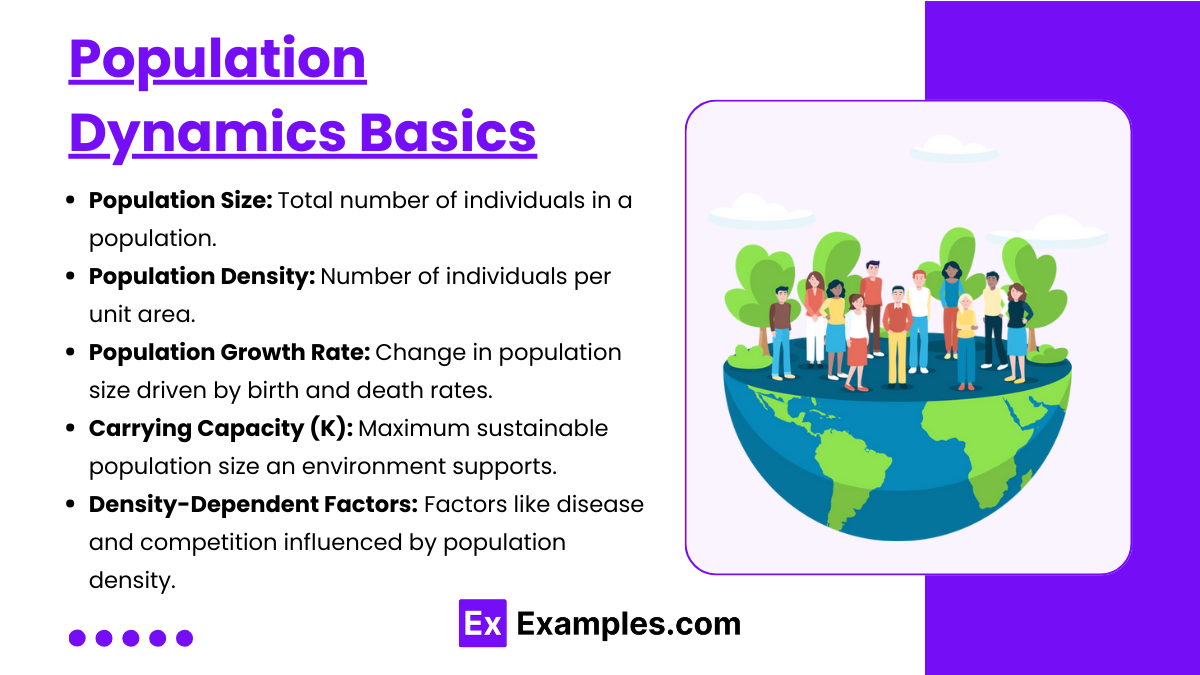
- Population Size: Total number of individuals within a population.
- Population Density: Number of individuals per unit area.
- Population Growth Rate: The rate at which the population size changes, driven by factors like birth rates and death rates.
- Carrying Capacity (K): The maximum population size that an environment can support sustainably.
- Density-Dependent Factors: Factors like disease and competition that affect populations based on their density.
- Density-Independent Factors: Factors like weather events that affect populations regardless of their density.
3. Evolution and Population Dynamics: How They Relate
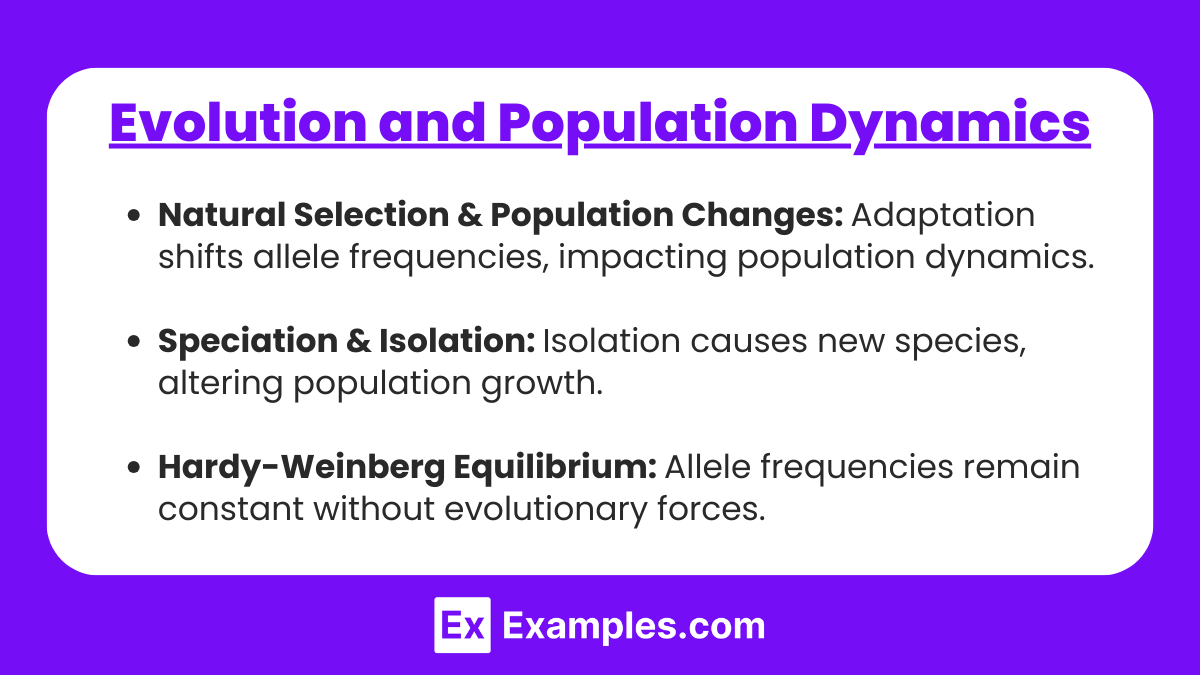
- Natural Selection and Population Changes:
- As populations adapt to their environments, allele frequencies shift, impacting population dynamics.
- Fitness (reproductive success) is crucial to understanding how evolutionary pressures drive population changes.
- Speciation and Population Isolation:
- Geographic or reproductive isolation can lead to speciation, affecting population dynamics.
- Genetic divergence occurs over time, leading to new populations that may follow different growth patterns.
- Hardy-Weinberg Equilibrium:
- This principle states that allele and genotype frequencies will remain constant in a population from generation to generation, provided that specific conditions are met (no mutation, no gene flow, random mating, etc.).
- It serves as a null hypothesis for detecting evolutionary changes in populations.
Examples
Example 1. Antibiotic Resistance in Bacteria
Bacteria can rapidly evolve through mutations and natural selection, especially in environments exposed to antibiotics. When antibiotics are introduced, only those bacteria with resistance genes survive and reproduce. Over time, this selective pressure causes the population to become predominantly resistant. As a result, the bacterial population dynamics shift towards higher survival and growth rates, making treatment more challenging and leading to changes in population size and genetic diversity.
Example 2. The Peppered Moth Evolution
During the Industrial Revolution in England, the peppered moth population exhibited a notable evolutionary shift. Initially, the light-colored variant was predominant, as it camouflaged well against lichen-covered trees. However, industrial soot darkened the tree bark, favoring the dark-colored variant through natural selection. The dark moths had a higher survival rate, changing the population dynamics by increasing their frequency. As air quality improved, the light-colored moths became more common again, demonstrating how environmental changes drive evolutionary responses and alter population dynamics.
Example 3. The Galápagos Finches and Adaptive Radiation
The Galápagos finches, observed by Charles Darwin, are a classic example of adaptive radiation, where a single ancestral species evolved into multiple species with diverse beak shapes. This evolution was driven by varying food resources on different islands, leading to different survival and reproductive advantages. Each finch species adapted to specific ecological niches, affecting population dynamics by diversifying feeding behaviors, competition levels, and population sizes within each niche.
Example 4. HIV Evolution and Host Population Dynamics
HIV, the virus that causes AIDS, is an example of rapid evolution within a host population. The virus mutates frequently, allowing it to adapt quickly to the host’s immune responses and drug treatments. As HIV evolves within individual hosts, its population dynamics shift, affecting viral load, transmission rates, and disease progression. This dynamic evolution complicates treatment efforts, as viral populations become resistant to antiretroviral therapies over time, necessitating changes in medical strategies and public health approaches.
Example 5. Invasive Species and Ecosystem Dynamics
When non-native species are introduced into new environments, they can undergo rapid evolution to adapt to different climates, food sources, and predators. For example, the introduction of rabbits in Australia led to rapid population growth due to a lack of natural predators and abundant food resources. The rabbits’ evolving characteristics, like increased reproductive rates, influenced local population dynamics, disrupting native species and causing ecological imbalances. This scenario demonstrates how invasive species can undergo evolutionary changes while significantly altering the population dynamics of ecosystems.
Practice Questions
Question 1
Which of the following mechanisms is primarily responsible for introducing new genetic variations into a population?
A) Natural Selection
B) Gene Flow
C) Mutation
D) Genetic Drift
Correct Answer: C) Mutation
Explanation:
Mutations are changes in the DNA sequence that create new genetic variations within a population. They are the original source of genetic diversity, allowing for different traits that can be acted upon by other evolutionary forces like natural selection. While natural selection, gene flow, and genetic drift modify existing genetic variations, mutation introduces entirely new alleles, making it a fundamental driver of evolution.
Question 2
What is the likely outcome when a small population undergoes a genetic bottleneck?
A) Increased genetic diversity
B) Decreased genetic diversity
C) Stabilized allele frequencies
D) Enhanced adaptability to new environments
Correct Answer: B) Decreased genetic diversity
Explanation:
A genetic bottleneck occurs when a population’s size is drastically reduced due to events like natural disasters or human activities. This reduction often leads to a loss of genetic diversity because only a small subset of the original population’s alleles is represented in the surviving group. With fewer alleles available, the population’s ability to adapt to new environments may be compromised, and the risk of inbreeding and extinction increases.
Question 3
How does gene flow affect the genetic diversity of populations?
A) It decreases genetic variation within a population.
B) It increases genetic variation between populations.
C) It reduces genetic differences between populations.
D) It leads to the complete isolation of populations.
Correct Answer: C) It reduces genetic differences between populations.
Explanation:
Gene flow is the transfer of alleles from one population to another, typically through migration or interbreeding. This process increases genetic diversity within a population by introducing new alleles and reduces genetic differences between populations by making their gene pools more similar. In contrast, isolation would prevent gene flow, allowing populations to diverge genetically over time.