Preparing for the MCAT involves mastering fat and protein metabolism, key topics in biochemistry. This area covers the breakdown, synthesis, and regulation of fats and proteins, focusing on processes such as beta-oxidation, lipogenesis, proteolysis, and transamination. It also includes understanding how fats and proteins contribute to ATP production and how they are regulated by hormones like insulin, glucagon, and cortisol. A solid understanding of fat and protein metabolism is essential for achieving a high MCAT score, as it relates to energy balance, cellular repair, and overall metabolic regulation.
Learning Objectives
When studying fat and protein metabolism for the MCAT, your learning objectives should include understanding the biochemical pathways involved in the breakdown and synthesis of fats and proteins, such as beta-oxidation, lipogenesis, proteolysis, and the urea cycle. Focus on how fatty acids are converted to ATP through beta-oxidation and how proteins are deaminated for energy or used in gluconeogenesis. Additionally, learn how hormones like insulin, glucagon, epinephrine, and cortisol regulate these processes during different metabolic states, such as fasting and feeding. Master the integration of fat and protein metabolism in overall energy homeostasis, their roles in cellular functions, and how they adapt under physiological stress conditions.
Fat Metabolism – Breakdown of Fats (Lipolysis)
Fat metabolism is the process of breaking down fats (lipids) into fatty acids and glycerol for energy.
It involves lipolysis, where stored fats are converted to free fatty acids.
These fatty acids undergo beta-oxidation to produce ATP, the body’s energy source.
Fat metabolism also leads to ketone production during prolonged fasting or low-carb intake.
It helps maintain energy balance, supports cell structure, and stores excess energy.
- Lipolysis occurs in the adipose tissue, breaking down triglycerides into free fatty acids (FFAs) and glycerol.
- Hormones like epinephrine and glucagon stimulate lipolysis, especially during fasting or prolonged exercise.
- FFAs are transported to the liver and muscles, where they undergo beta-oxidation to generate energy.
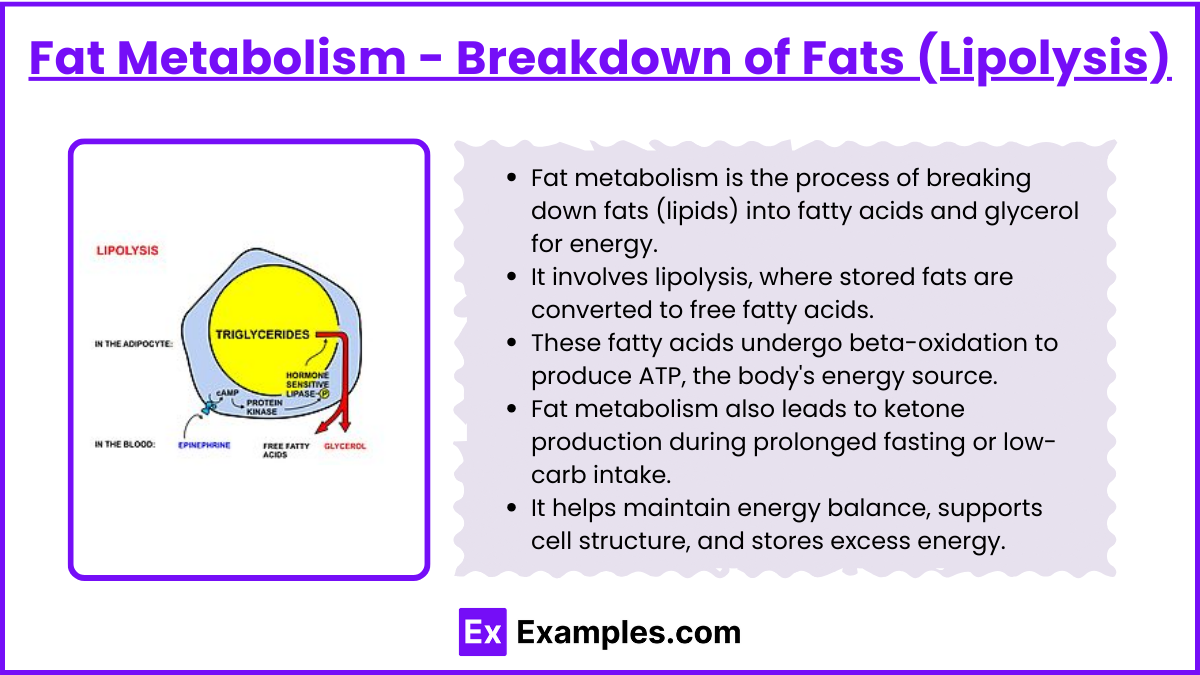
1. Beta-Oxidation of Fatty Acids
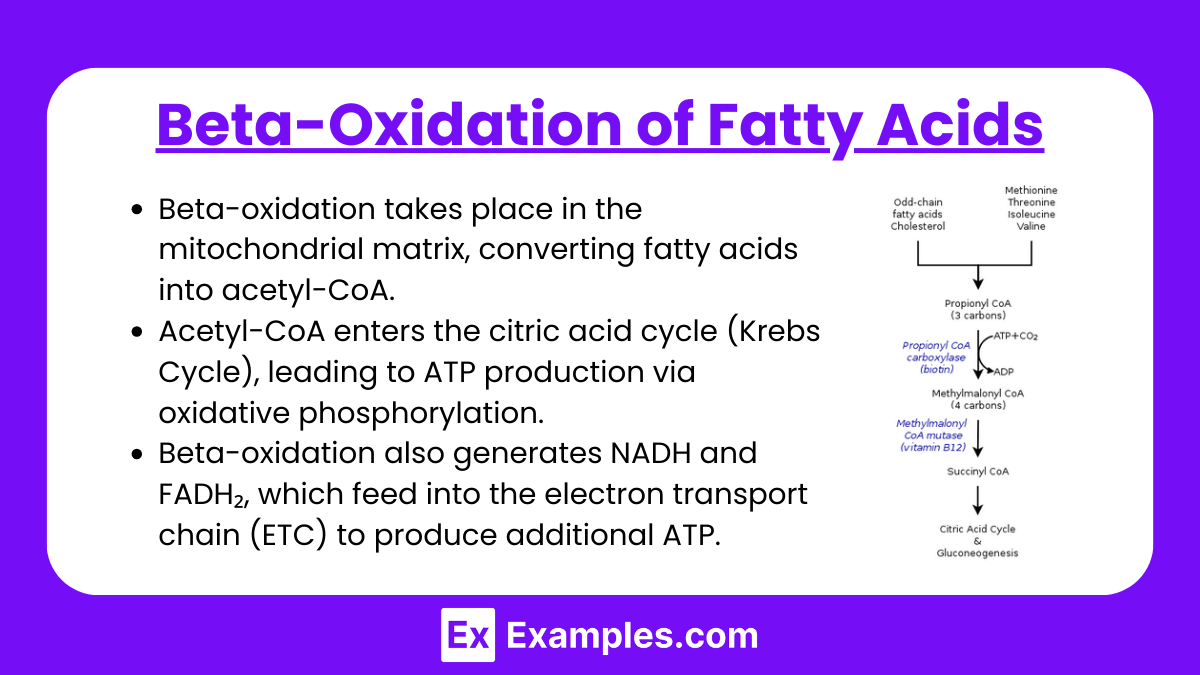
- Beta-oxidation takes place in the mitochondrial matrix, converting fatty acids into acetyl-CoA.
- Acetyl-CoA enters the citric acid cycle (Krebs Cycle), leading to ATP production via oxidative phosphorylation.
- Beta-oxidation also generates NADH and FADH₂, which feed into the electron transport chain (ETC) to produce additional ATP.
2. Ketogenesis
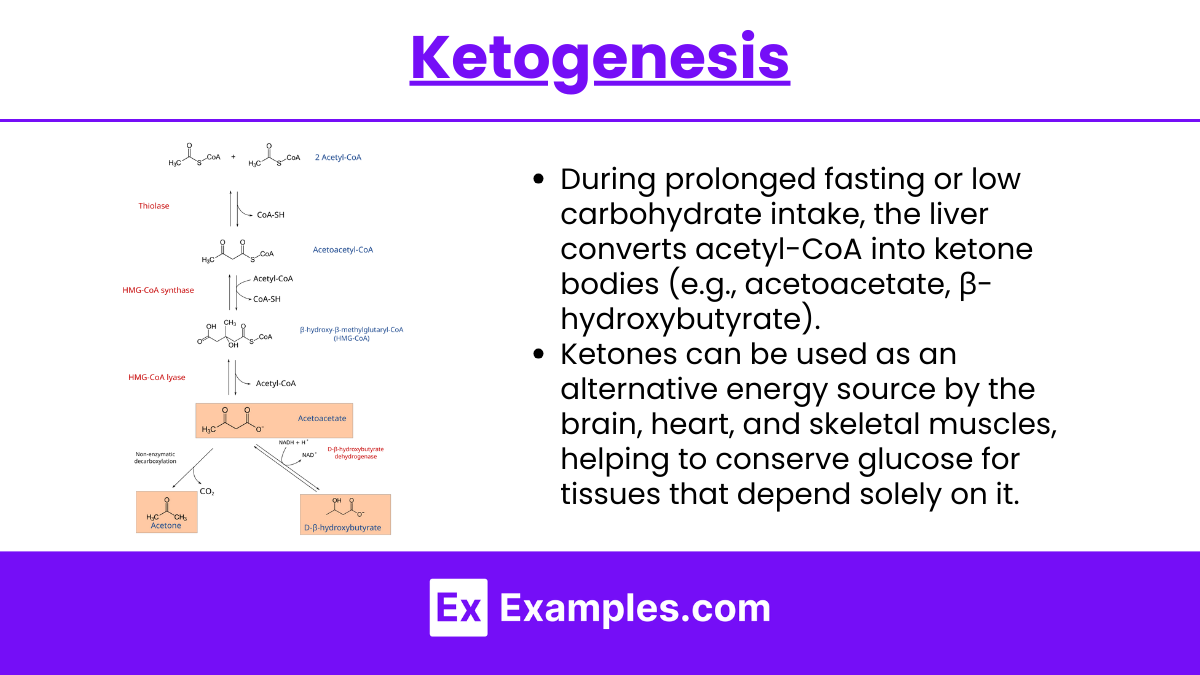
- During prolonged fasting or low carbohydrate intake, the liver converts acetyl-CoA into ketone bodies (e.g., acetoacetate, β-hydroxybutyrate).
- Ketones can be used as an alternative energy source by the brain, heart, and skeletal muscles, helping to conserve glucose for tissues that depend solely on it.
3. Regulation of Fat Metabolism
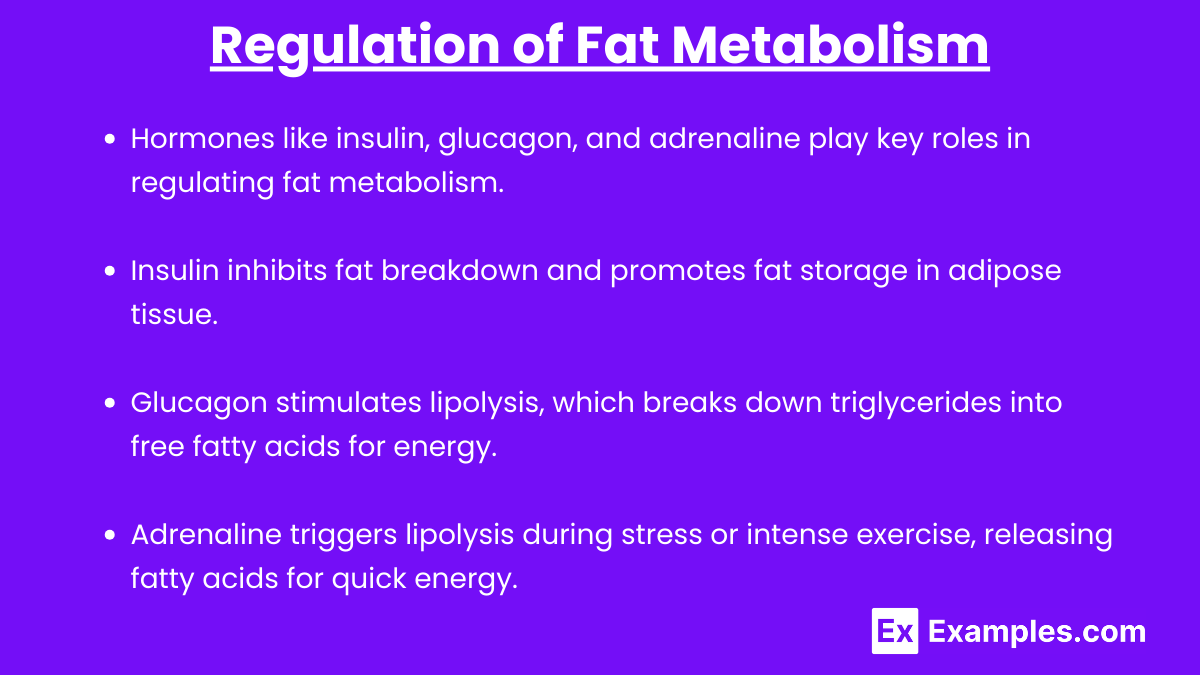
- Hormones like insulin, glucagon, and adrenaline play key roles in regulating fat metabolism.
- Insulin inhibits fat breakdown and promotes fat storage in adipose tissue.
- Glucagon stimulates lipolysis, which breaks down triglycerides into free fatty acids for energy.
- Adrenaline triggers lipolysis during stress or intense exercise, releasing fatty acids for quick energy.
- Hormone-sensitive lipase (HSL) is activated to mobilize stored fat during energy demand.
- Perilipin proteins in adipose cells regulate access to stored fat by controlling lipase activity.
- Acetyl-CoA is formed from fatty acids during beta-oxidation in the mitochondria.
- Beta-oxidation produces NADH and FADH2, which feed into the electron transport chain to generate ATP.
- The liver converts excess fatty acids into ketone bodies during prolonged fasting or low-carb diets.
- The process of ketogenesis provides an alternative energy source, especially for the brain and muscles.
- The regulation of fat metabolism is crucial for maintaining energy balance and preventing metabolic disorders like obesity or diabetes.
Protein Metabolism
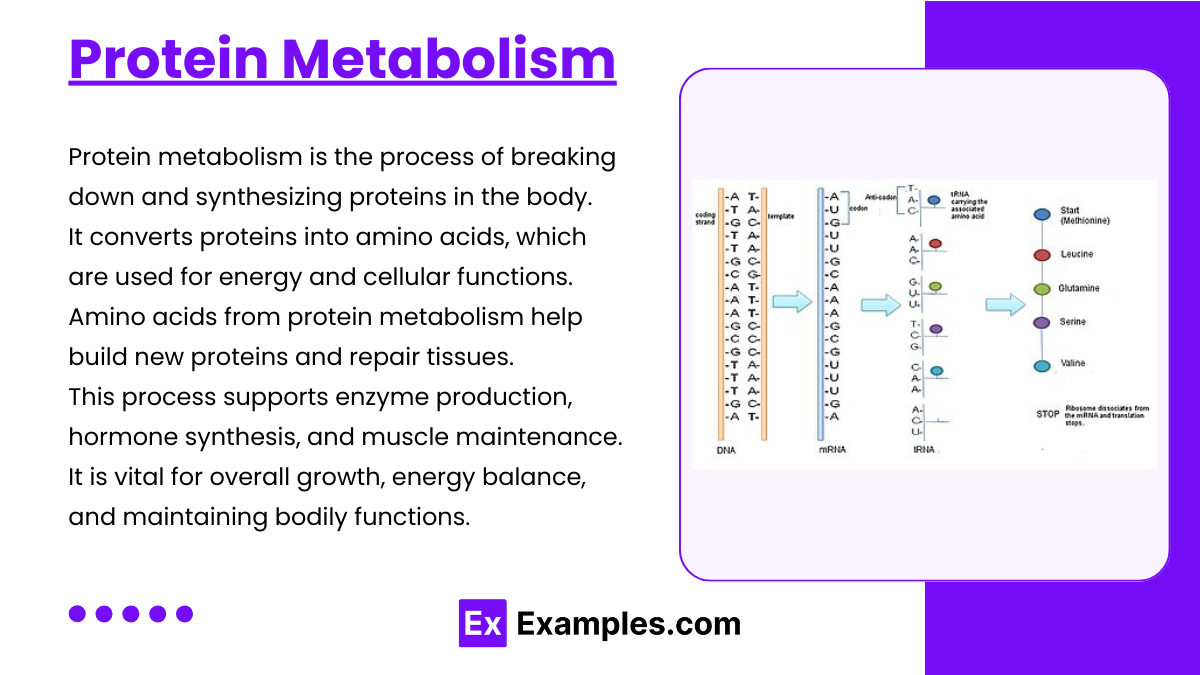
Protein metabolism is the breakdown and synthesis of proteins into amino acids, which are used for energy production, building new proteins, and other bodily functions. It ensures the body maintains muscle mass, produces enzymes, and supports other vital processes.
1. Protein Catabolism
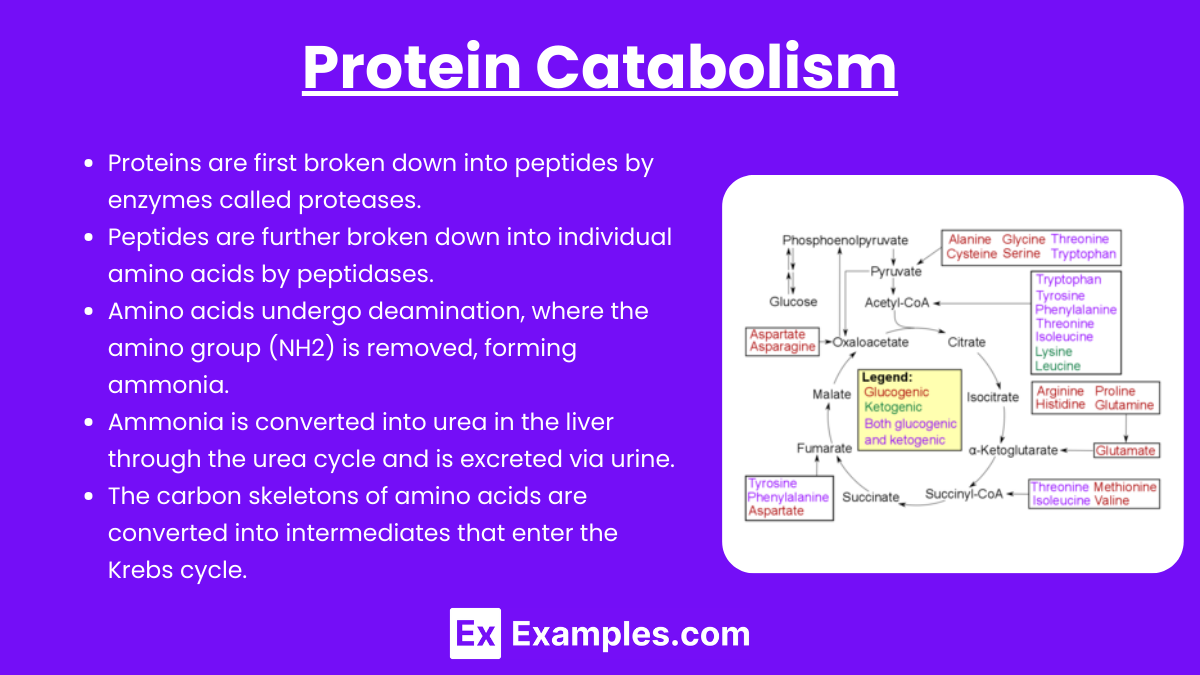
Protein catabolism is the process by which proteins are broken down into amino acids, which can be used for energy production or converted into other compounds. It becomes a critical energy source when carbohydrate and fat stores are low, such as during prolonged fasting or intense physical stress. The breakdown of proteins also plays a role in recycling amino acids for new protein synthesis.
- Proteins are first broken down into peptides by enzymes called proteases.
- Peptides are further broken down into individual amino acids by peptidases.
- Amino acids undergo deamination, where the amino group (NH2) is removed, forming ammonia.
- Ammonia is converted into urea in the liver through the urea cycle and is excreted via urine.
- The carbon skeletons of amino acids are converted into intermediates that enter the Krebs cycle.
2. Urea Cycle
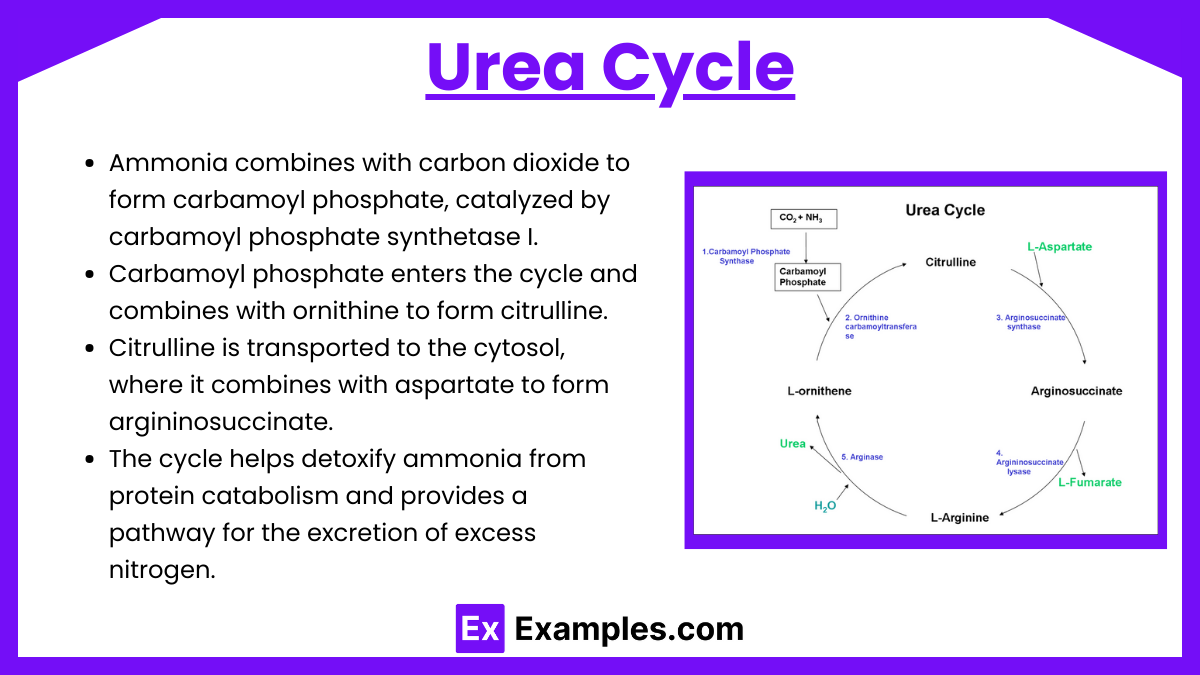
The urea cycle, also known as the ornithine cycle, is a metabolic pathway that occurs primarily in the liver. Its main function is to convert toxic ammonia, produced during amino acid breakdown, into urea, which is less toxic and can be safely excreted via the urine. This cycle is crucial for maintaining nitrogen balance and preventing the buildup of harmful levels of ammonia in the body.
- Ammonia combines with carbon dioxide to form carbamoyl phosphate, catalyzed by carbamoyl phosphate synthetase I.
- Carbamoyl phosphate enters the cycle and combines with ornithine to form citrulline.
- Citrulline is transported to the cytosol, where it combines with aspartate to form argininosuccinate.
- Argininosuccinate is split into arginine and fumarate by the enzyme argininosuccinate lyase.
- Arginine is then hydrolyzed by arginase to release urea and regenerate ornithine, completing the cycle.
- Urea, formed in the liver, is transported to the kidneys for excretion via urine.
- The cycle is regulated by substrate availability and enzyme activity, particularly carbamoyl phosphate synthetase I.
- Hormones like glucagon enhance urea cycle activity during protein-rich meals or prolonged fasting.
- The cycle helps detoxify ammonia from protein catabolism and provides a pathway for the excretion of excess nitrogen.
3. Regulation of Protein Metabolism
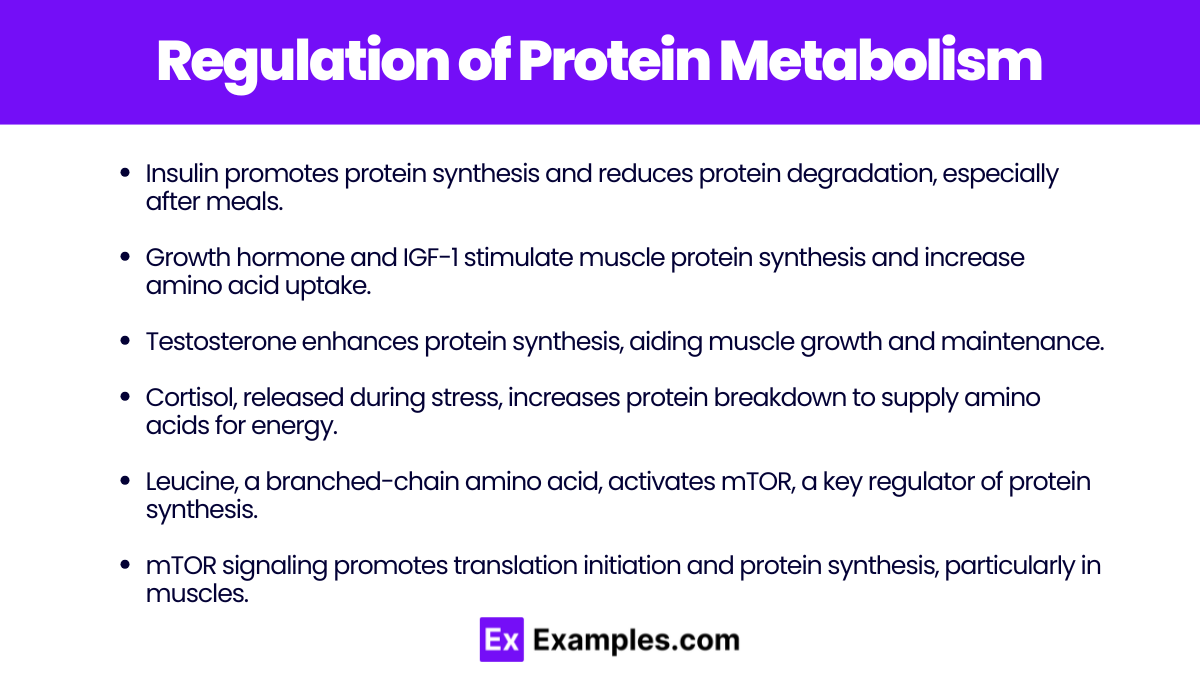
- Insulin promotes protein synthesis and reduces protein degradation, especially after meals.
- Growth hormone and IGF-1 stimulate muscle protein synthesis and increase amino acid uptake.
- Testosterone enhances protein synthesis, aiding muscle growth and maintenance.
- Cortisol, released during stress, increases protein breakdown to supply amino acids for energy.
- Leucine, a branched-chain amino acid, activates mTOR, a key regulator of protein synthesis.
- mTOR signaling promotes translation initiation and protein synthesis, particularly in muscles.
- AMPK, another enzyme, inhibits protein synthesis during energy stress, favoring catabolism.
- Glucagon increases during fasting, stimulating amino acid release from muscle for gluconeogenesis.
- The liver regulates amino acid metabolism, converting them into glucose, ketones, or other compounds.
- Dietary protein intake influences metabolism, with high intake promoting synthesis and low intake increasing degradation.
Examples
Example 1. Prolonged Fasting
During prolonged fasting, the body primarily relies on fat metabolism to produce energy. As glycogen stores deplete, triglycerides stored in adipose tissues are broken down into fatty acids and glycerol. Fatty acids undergo beta-oxidation to generate ATP, while glycerol can be used in gluconeogenesis to maintain blood glucose levels. When fasting continues, ketone bodies are produced via ketogenesis, providing an alternative energy source, particularly for the brain, which is less reliant on glucose.
Example 2. Endurance Exercise
During long-duration, low-to-moderate intensity exercises like marathon running, the body shifts to fat metabolism for sustained energy production. Muscle cells utilize fatty acids through beta-oxidation to generate ATP. As exercise duration increases, the body’s dependence on fat as a fuel source increases, preserving glycogen stores and maintaining energy levels. Additionally, protein metabolism may play a role in producing glucose via gluconeogenesis, using amino acids as substrates when carbohydrate reserves are low.
Example 3. Low-Carbohydrate, High-Fat Diet (Ketogenic Diet)
In a ketogenic diet, the body adapts to using fats as its primary energy source due to restricted carbohydrate intake. This shift induces the liver to increase ketogenesis, producing ketone bodies that provide energy to various organs, including the brain and muscles. Protein metabolism is also critical in such a diet, as amino acids contribute to gluconeogenesis, ensuring adequate glucose supply for tissues that cannot rely on fats or ketones.
Example 4. Starvation State
In a state of starvation, the body maximizes fat and protein metabolism to preserve life. Initially, fats become the primary energy source, undergoing lipolysis and beta-oxidation to provide ATP. As starvation continues, protein metabolism becomes more significant, with muscle proteins being broken down into amino acids, which are then converted into glucose through gluconeogenesis. This process helps maintain vital organ functions, especially in glucose-dependent tissues like the brain and red blood cells.
Example 5. High-Intensity Interval Training (HIIT)
During HIIT workouts, the body initially uses stored glycogen for rapid energy production. As the workout continues and glycogen depletes, the body starts to utilize fat metabolism, breaking down triglycerides into fatty acids for ATP production. Protein metabolism may also become involved, particularly in the post-exercise recovery phase, where amino acids are used to repair muscle tissue and replenish energy through gluconeogenesis, supporting overall muscle growth and energy recovery.
Practice Questions
Question 1
Which of the following best describes the process of beta-oxidation?
A) Conversion of amino acids into glucose.
B) Breakdown of fatty acids into acetyl-CoA for energy production.
C) Conversion of glucose into pyruvate in the cytosol.
D) Synthesis of triglycerides in the liver for storage.
Correct Answer: B) Breakdown of fatty acids into acetyl-CoA for energy production.
Explanation:
Beta-oxidation is the process by which fatty acids are broken down in the mitochondria to form acetyl-CoA. Acetyl-CoA then enters the citric acid cycle, where it contributes to the production of ATP. This process is crucial for energy production, especially during prolonged exercise, fasting, or low-carbohydrate intake. Options A and C relate to glucose metabolism, while option D involves fat synthesis, not breakdown.
Question 2
During prolonged fasting, which of the following metabolic changes primarily occurs?
A) Increased glycogen storage in the liver.
B) Decreased lipolysis in adipose tissues.
C) Increased ketone production by the liver.
D) Increased protein synthesis in muscle cells.
Correct Answer: C) Increased ketone production by the liver.
Explanation:
During prolonged fasting, the liver increases the production of ketone bodies from acetyl-CoA derived from fatty acid oxidation. This shift occurs as the body adapts to using fats for energy when glycogen stores are depleted. Ketones serve as an alternative energy source, particularly for the brain. Option A is incorrect because glycogen is depleted rather than stored. Option B is incorrect because lipolysis increases to mobilize fatty acids. Option D is incorrect because protein catabolism, not synthesis, increases to provide substrates for gluconeogenesis.
Question 3
Which of the following roles does protein metabolism primarily serve during gluconeogenesis?
A) Providing substrates to synthesize fatty acids.
B) Converting acetyl-CoA into glucose for immediate energy.
C) Producing amino acids that can be converted to glucose.
D) Directly generating ATP through the electron transport chain.
Correct Answer: C) Producing amino acids that can be converted to glucose.
Explanation:
During gluconeogenesis, certain amino acids (glucogenic amino acids) are converted into intermediates that can be transformed into glucose. This process is especially important during fasting or when carbohydrate intake is low, helping maintain blood glucose levels for tissues that depend on glucose. Option A is incorrect because protein metabolism does not synthesize fatty acids. Option B is incorrect since acetyl-CoA cannot be converted back to glucose. Option D is incorrect because protein metabolism does not directly generate ATP in the electron transport chain; it supplies substrates for gluconeogenesis instead.