Foundation 4 of the MCAT assesses your understanding of the chemical and physical principles that underpin biological systems. This section requires knowledge of key concepts like thermodynamics, fluid dynamics, electrochemistry, and molecular interactions. Mastery of these principles enables you to analyze and interpret passages, applying them to biological processes and experimental data critical for success on the MCAT.
Learning Objective
In studying “Foundation 4: Physical Processes: Chemical and Physical Foundations of Biological Systems” for the MCAT, you should learn to apply principles of physics and chemistry to biological systems, including concepts such as fluid dynamics, thermodynamics, and electrostatics. Analyze how forces, energy, and motion relate to biological structures and processes. Understand the role of chemical bonds, reaction rates, and equilibrium in living organisms. Evaluate how physical principles explain physiological processes such as blood flow, gas exchange, and nerve signaling. Practice applying these concepts through MCAT-style passages, integrating problem-solving and critical thinking.
1. Chemical Principles in Biological Systems
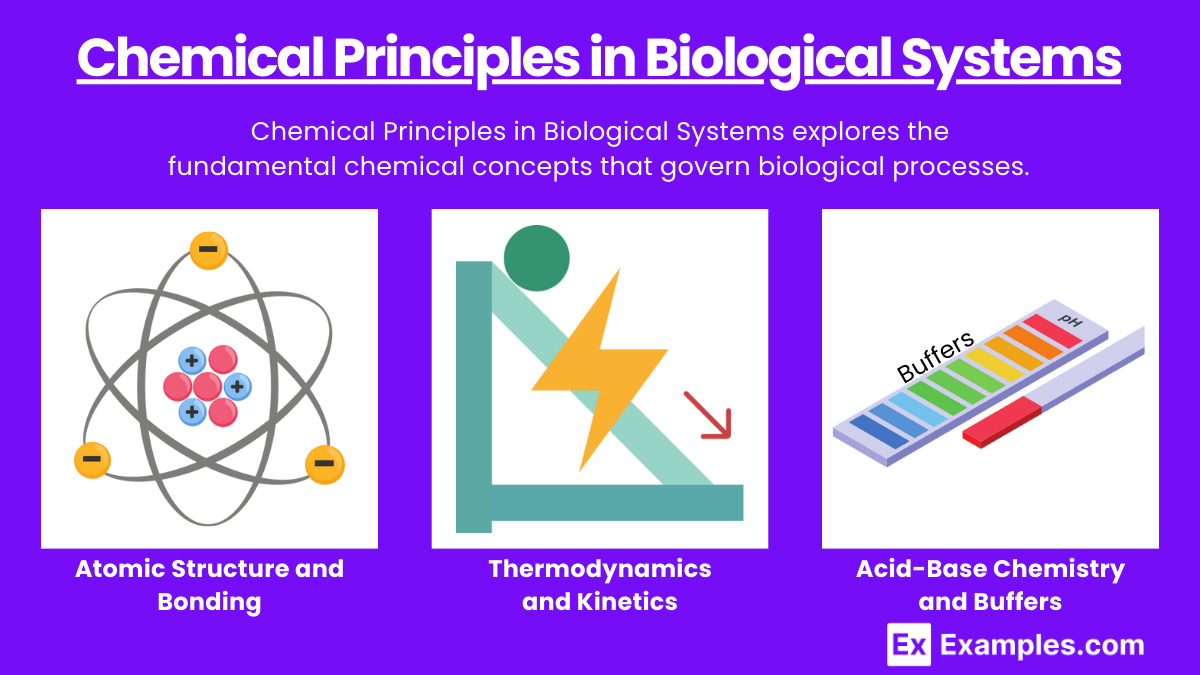
- Atomic Structure and Bonding: Know how electron configurations influence reactivity, particularly in biological molecules. Understand covalent, ionic, and hydrogen bonding, especially as it relates to water, proteins, and nucleic acids.
- Thermodynamics and Kinetics: Review concepts like Gibbs free energy, enthalpy, entropy, and activation energy. Focus on how these principles drive biological processes, including enzyme activity and biochemical pathways.
- Acid-Base Chemistry and Buffers: Master pH, pKa, and the Henderson-Hasselbalch equation. Study how buffers function in maintaining homeostasis, with examples like the bicarbonate buffer system in blood.
2. Physical Principles Applied to Biological Systems
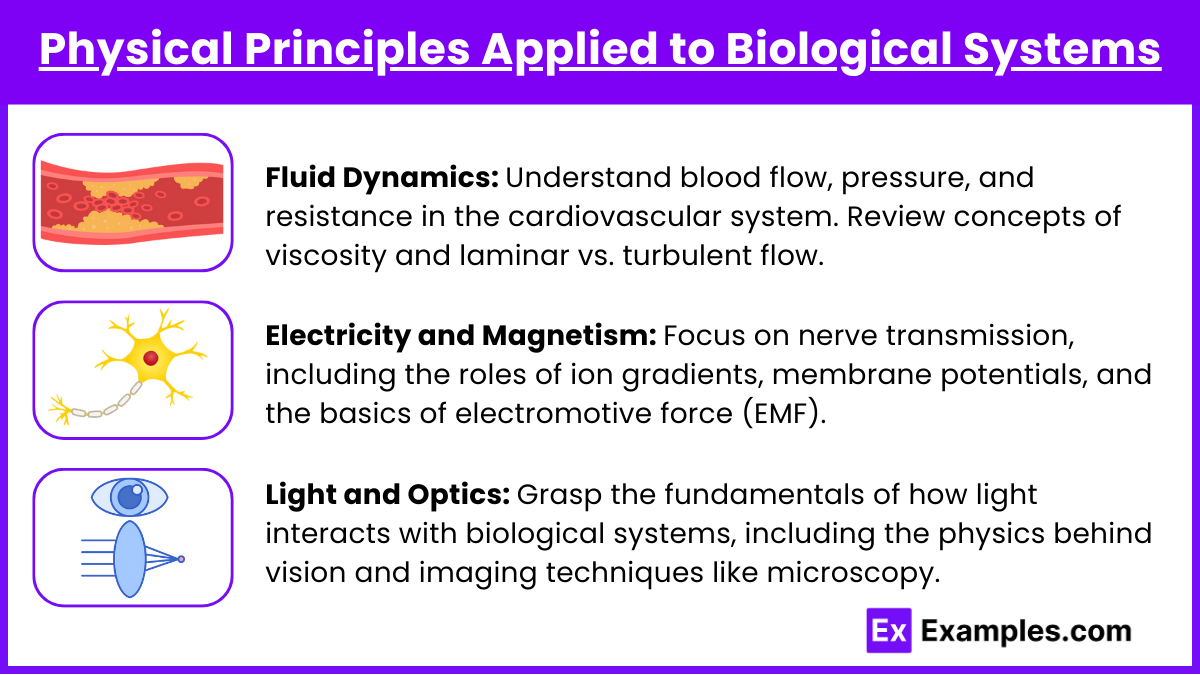
- Fluid Dynamics: Understand blood flow, pressure, and resistance in the cardiovascular system. Review concepts of viscosity and laminar vs. turbulent flow.
- Electricity and Magnetism: Focus on nerve transmission, including the roles of ion gradients, membrane potentials, and the basics of electromotive force (EMF).
- Light and Optics: Grasp the fundamentals of how light interacts with biological systems, including the physics behind vision and imaging techniques like microscopy.
3. Fluid Dynamics and Blood Flow
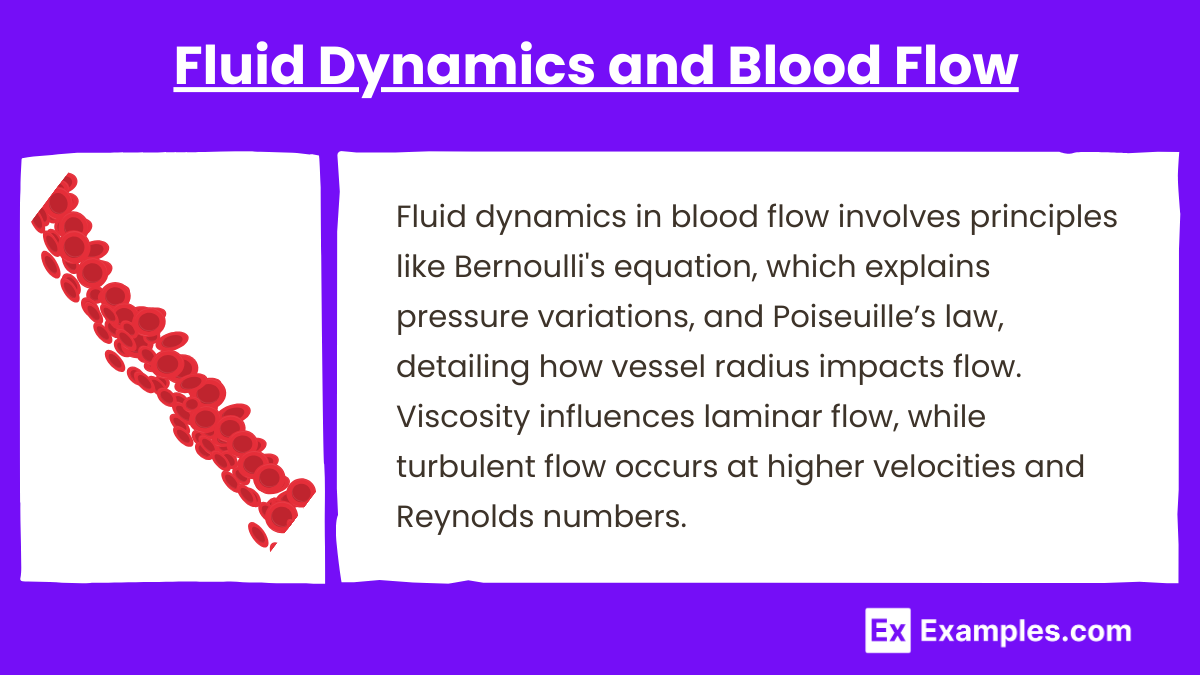
Fluid dynamics in blood flow involves principles like Bernoulli’s equation, which explains pressure variations, and Poiseuille’s law, detailing how vessel radius impacts flow. Viscosity influences laminar flow, while turbulent flow occurs at higher velocities and Reynolds numbers.
- Bernoulli’s Equation: Apply this principle to understand blood flow and pressures in the circulatory system.
- Viscosity and Laminar Flow: Comprehend how viscosity affects blood flow, and the impact of Reynolds number in determining laminar vs. turbulent flow.
- Poiseuille’s Law: Examine how blood vessel radius and length affect resistance and flow rates.
4. Energy, Work, and Biological Processes
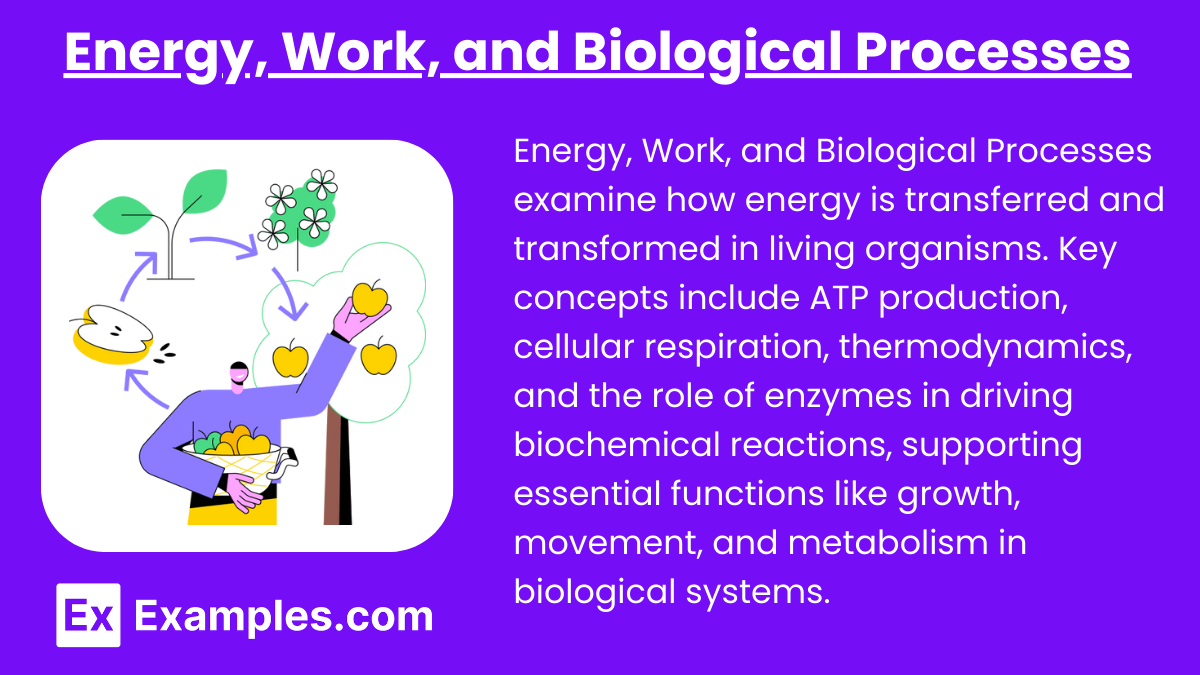
Energy, Work, and Biological Processes examine how energy is transferred and transformed in living organisms. Key concepts include ATP production, cellular respiration, thermodynamics, and the role of enzymes in driving biochemical reactions, supporting essential functions like growth, movement, and metabolism in biological systems.
- Principles of Bioenergetics: Focus on ATP as an energy currency, the concept of coupled reactions, and the importance of metabolic pathways like glycolysis, the citric acid cycle, and oxidative phosphorylation.
- Molecular Transport Mechanisms: Understand diffusion, osmosis, active transport, and facilitated transport. Review the role of channels, pumps, and carrier proteins in cellular transport.
Examples
Example 1: Enzyme Catalysis and Kinetics
In this passage, analyze how changes in substrate concentration affect enzyme reaction rates. Apply Michaelis-Menten kinetics to determine the enzyme’s efficiency and the impact of inhibitors. Expect to interpret data from reaction rate experiments and understand the roles of competitive and non-competitive inhibition.
Example 2: Gas Exchange and Blood Transport
This example focuses on the role of partial pressures in oxygen and carbon dioxide transport. You’ll explore how hemoglobin binding affinities change with pH and CO2 levels (Bohr effect), affecting oxygen delivery in tissues. Interpretation of gas exchange diagrams may be required.
Example 3: Thermodynamics in Cellular Respiration
Analyze how Gibbs free energy changes drive reactions in cellular respiration. Calculate energy yields from glucose breakdown and evaluate how ATP production is linked to electron transport and chemiosmosis. Passage questions may include interpreting reaction coupling and understanding the efficiency of ATP synthesis.
Example 4: Fluid Flow in Blood Vessels
In this passage, understand how Poiseuille’s law applies to blood flow, addressing factors like vessel radius and pressure. Analyze how these variables affect circulation and implications for cardiovascular health. Expect calculations on resistance, flow rate, and the effects of viscosity on blood movement.
Example 5: Electrolyte Balance and Nerve Impulses
This passage explores how ion gradients across neuron membranes create action potentials. You’ll examine the role of sodium and potassium ions in membrane depolarization and repolarization, assessing how changes in ion channels influence nerve signal transmission. Passage may involve interpreting graphs of action potential phases.
Practice Questions
Question 1:
Which of the following best describes how hemoglobin’s oxygen-binding affinity changes in response to increased CO₂ concentration?
A) Hemoglobin’s affinity for oxygen increases, promoting oxygen retention in tissues.
B) Hemoglobin’s affinity for oxygen decreases, facilitating oxygen release in tissues.
C) Hemoglobin’s affinity for oxygen remains unchanged, as CO₂ does not affect binding.
D) Hemoglobin’s affinity for oxygen initially increases but decreases at high CO₂ levels.
Answer: B) Hemoglobin’s affinity for oxygen decreases, facilitating oxygen release in tissues.
Explanation:
Increased CO₂ concentration in the blood leads to the formation of carbonic acid, which dissociates into hydrogen ions, lowering pH. This induces the Bohr effect, where hemoglobin’s affinity for oxygen decreases in acidic environments, promoting oxygen release in tissues with higher CO₂ concentrations.
Question 2:
A reaction has a ΔG° of -25 kJ/mol. Which of the following statements best describes this reaction?
A) The reaction is endergonic and non-spontaneous.
B) The reaction is exergonic and spontaneous.
C) The reaction requires an input of energy to proceed.
D) The reaction has reached equilibrium and cannot proceed.
Answer: B) The reaction is exergonic and spontaneous.
Explanation:
A negative ΔG° value indicates that the reaction is exergonic and releases free energy. Such reactions are spontaneous, meaning they can proceed without an input of energy. Endergonic reactions, which require energy, have positive ΔG° values, and equilibrium implies ΔG° = 0.
Question 3:
During an action potential, what is the primary cause of depolarization in a neuron?
A) Outflow of sodium (Na⁺) ions from the neuron
B) Inflow of potassium (K⁺) ions into the neuron
C) Inflow of sodium (Na⁺) ions into the neuron
D) Outflow of chloride (Cl⁻) ions from the neuron
Answer: C) Inflow of sodium (Na⁺) ions into the neuron
Explanation:
During depolarization, voltage-gated sodium channels open, allowing Na⁺ ions to flow into the neuron. This influx of positive ions causes the membrane potential to become more positive, initiating the action potential. Potassium ions are involved in repolarization, where K⁺ exits the neuron to restore resting potential.