The MCAT’s Foundation 5 section examines essential chemical and physical principles applied to biological systems. Covering topics like thermodynamics, kinetics, and acid-base chemistry, this section tests your ability to analyze biological reactions and processes. Practice passages focus on interpreting data, understanding chemical interactions, and applying scientific reasoning, essential for excelling in the Chemical and Physical Foundations of Biological Systems section.
Learning Objective
In studying “Foundation 5: Chemical Processes: Chemical and Physical Foundations of Biological Systems” for the MCAT, you should learn to understand the key chemical processes that govern biological systems, including acid-base chemistry, oxidation-reduction reactions, and reaction kinetics. Analyze the structure, function, and behavior of biomolecules, such as proteins, carbohydrates, lipids, and nucleic acids. Evaluate how chemical reactions contribute to metabolic pathways, energy production, and enzyme function. Additionally, explore concepts related to molecular interactions, equilibrium, and thermodynamics, and apply this knowledge to practice passages that test your problem-solving and application skills in a biological context.
1. Chemical Reactions and Stoichiometry
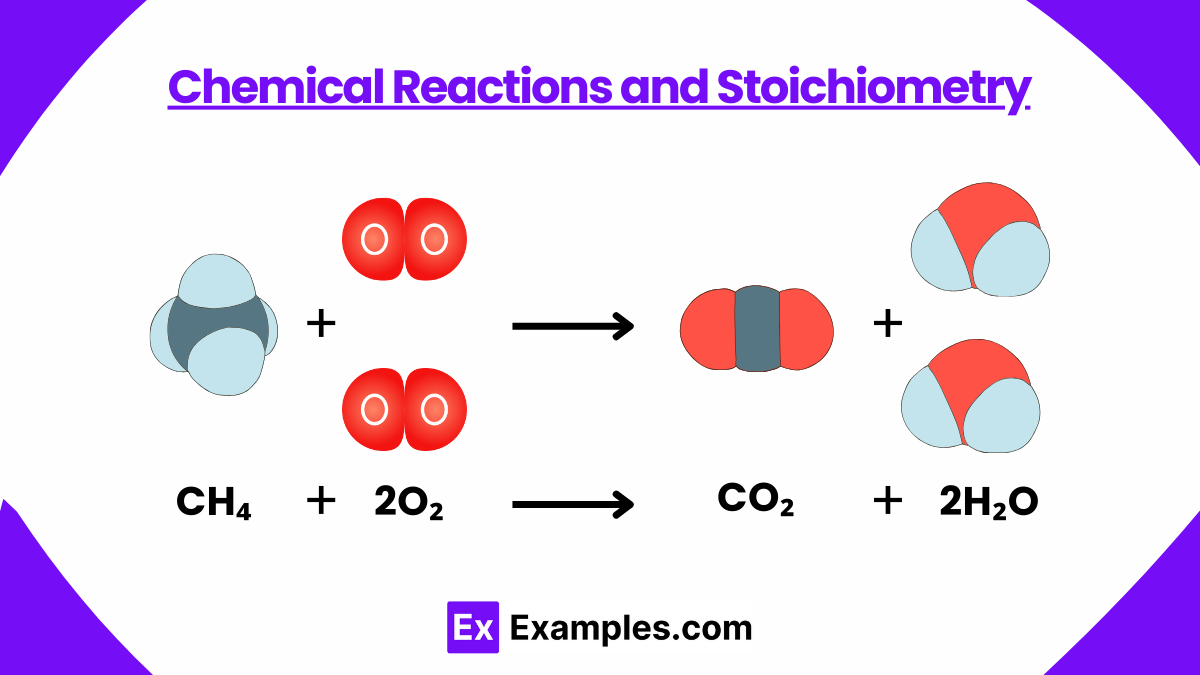
- Reaction Types: Be familiar with synthesis, decomposition, single replacement, double replacement, and combustion reactions.
- Balancing Equations: Practice balancing chemical equations, as this is crucial for understanding reaction stoichiometry.
- Stoichiometric Calculations: Grasp mole-to-mole ratios, limiting reagents, and percent yield calculations.
- Thermodynamics: Understand enthalpy (ΔH), entropy (ΔS), and Gibbs free energy (ΔG), particularly in biological reactions and energy transformations within cells.
2. Acid-Base Chemistry
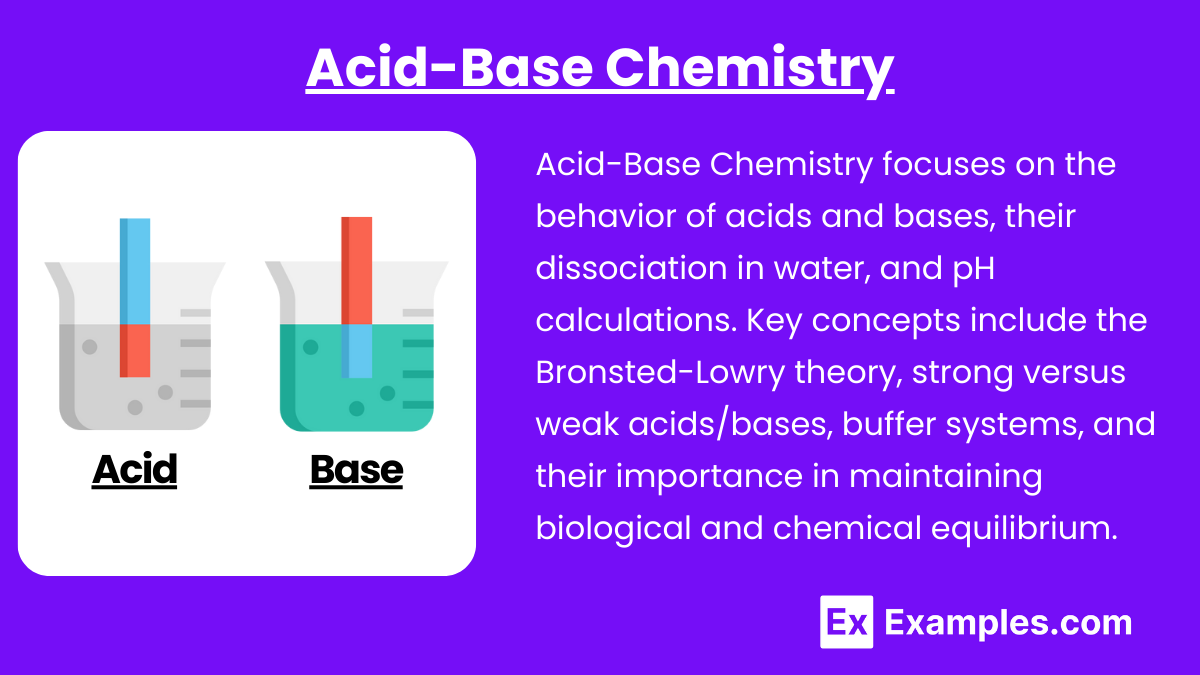
- Acid and Base Definitions: Know Arrhenius, Bronsted-Lowry, and Lewis definitions.
- pH, pKa, and pOH: Be able to calculate and interpret pH and pOH, especially in buffering systems.
- Buffer Systems: Understand how buffers work in biological systems, including the bicarbonate buffer system in blood.
- Titration Curves: Familiarize yourself with interpreting titration curves and identifying equivalence points, which is especially important for understanding enzyme activity and drug efficacy.
3. Electrochemistry
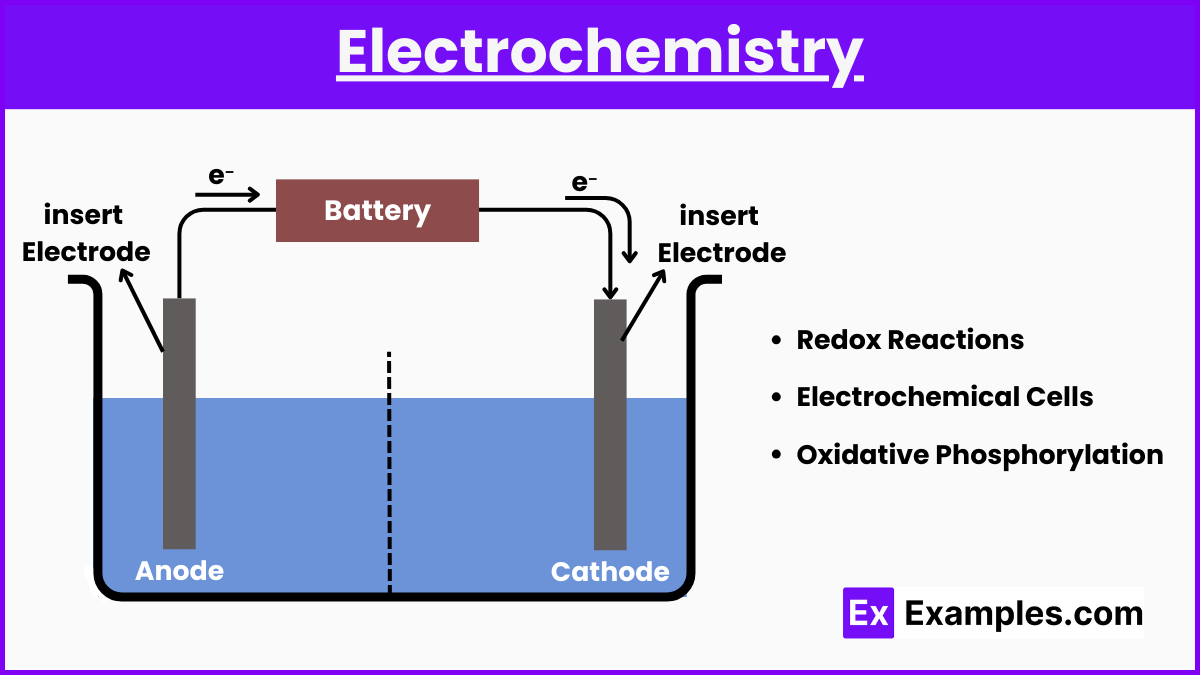
- Redox Reactions: Be able to identify oxidation and reduction, and understand the role of redox reactions in biological systems like cellular respiration.
- Electrochemical Cells: Understand galvanic (voltaic) and electrolytic cells, including cell potential and the Nernst equation.
- Oxidative Phosphorylation: Know how the electron transport chain in mitochondria functions as an electrochemical gradient driving ATP synthesis.
4. Fluid Dynamics and Gas Laws
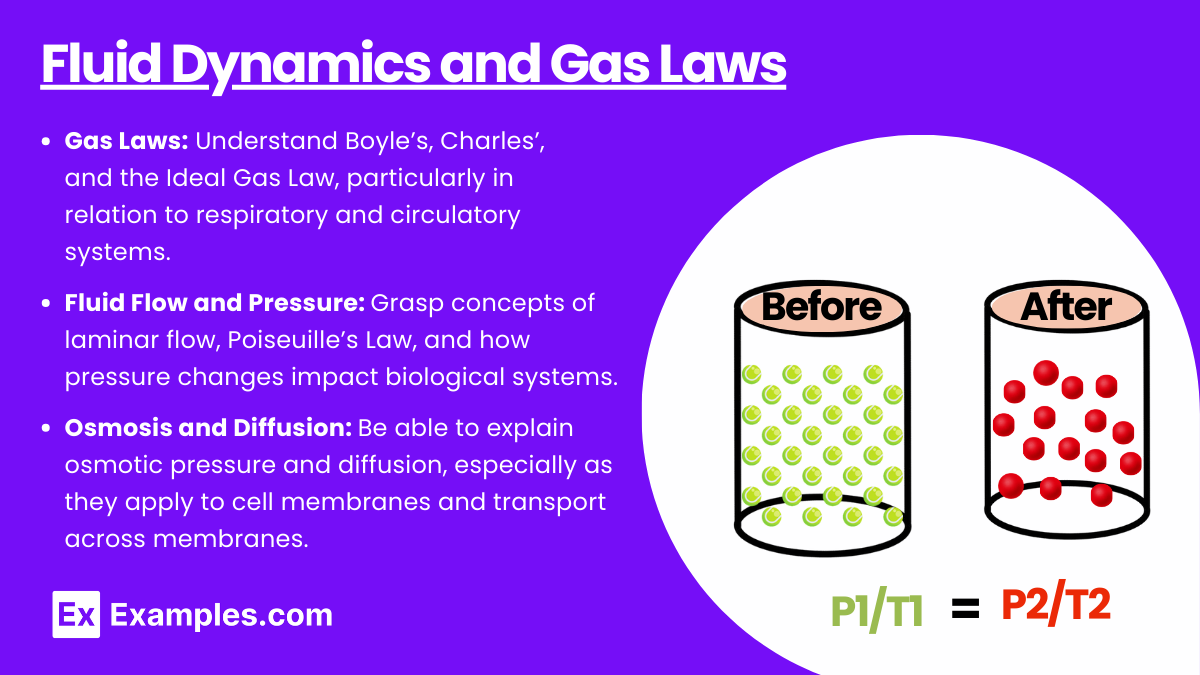
- Gas Laws: Understand Boyle’s, Charles’, and the Ideal Gas Law, particularly in relation to respiratory and circulatory systems.
- Fluid Flow and Pressure: Grasp concepts of laminar flow, Poiseuille’s Law, and how pressure changes impact biological systems (e.g., blood pressure, air flow in lungs).
- Osmosis and Diffusion: Be able to explain osmotic pressure and diffusion, especially as they apply to cell membranes and transport across membranes.
Examples
Example 1: Enzyme Kinetics and Inhibition
A passage describing an experiment testing the effects of competitive and non-competitive inhibitors on enzyme activity, illustrating Michaelis-Menten kinetics. Understanding how inhibitors impact reaction rates and enzyme-substrate affinity is crucial for interpreting experimental results and predicting biological responses.
Example 2: Thermodynamics in Metabolic Reactions
This passage covers glycolysis and Krebs cycle energetics, requiring an understanding of enthalpy, entropy, and Gibbs free energy. By analyzing energy transformations, you interpret whether metabolic pathways are spontaneous or require energy input, vital for understanding cellular bioenergetics.
Example 3: Acid-Base Balance in the Blood
A passage on the bicarbonate buffer system explores how blood pH is regulated. It challenges you to calculate pH changes using Henderson-Hasselbalch equation, reflecting physiological responses to respiratory and metabolic disturbances in pH, essential in medical contexts.
Example 4: Fluid Dynamics in Circulatory Systems
This passage involves calculating blood flow and pressure using Poiseuille’s Law. Understanding how vessel radius and viscosity affect flow rates enables you to predict physiological effects in scenarios like atherosclerosis, where blood flow dynamics are altered due to narrowing arteries.
Example 5: Gas Exchange and Partial Pressures
A passage describes how oxygen and carbon dioxide exchange across alveolar membranes. Applying Dalton’s Law and principles of diffusion, you predict gas movement in different environmental conditions, essential for understanding respiratory physiology and gas transport efficiency in biological systems.
Practice Questions
Question 1:
An enzyme catalyzes the conversion of substrate X to product Y. Which of the following changes is expected to occur if a competitive inhibitor is introduced into the reaction?
A) The maximum reaction rate (Vmax) decreases, and the Michaelis constant (Km) remains unchanged.
B) Both Vmax and Km decrease.
C) Vmax remains unchanged, and Km increases.
D) Both Vmax and Km increase.
Answer: C) Vmax remains unchanged, and Km increases.
Explanation:
A competitive inhibitor binds to the enzyme’s active site, preventing substrate X from binding. This increases the Km (substrate concentration required to reach half of Vmax), indicating reduced enzyme-substrate affinity. However, Vmax remains unchanged because adding more substrate can overcome the inhibition by outcompeting the inhibitor.
Question 2:
A patient experiences respiratory alkalosis, characterized by an elevated blood pH due to hyperventilation. Which of the following compensatory mechanisms is most likely to occur to restore blood pH to normal?
A) The kidneys increase excretion of bicarbonate (HCO₃⁻).
B) The kidneys increase retention of bicarbonate (HCO₃⁻).
C) The lungs decrease ventilation rate.
D) The lungs increase carbon dioxide exhalation.
Answer: A) The kidneys increase excretion of bicarbonate (HCO₃⁻).
Explanation:
Respiratory alkalosis occurs due to excessive loss of CO₂ from hyperventilation, raising blood pH. To compensate, the kidneys excrete bicarbonate to reduce pH and bring it back to normal. Decreasing ventilation (Option C) could help, but it’s an involuntary response and not a compensatory mechanism in this scenario.
Question 3:
In a closed system, a sample of an ideal gas undergoes compression, reducing its volume while maintaining a constant temperature. According to the ideal gas law, which of the following is true about the gas?
A) Its pressure decreases.
B) Its pressure increases.
C) Its number of moles decreases.
D) Its temperature decreases.
Answer: B) Its pressure increases.
Explanation:
The ideal gas law is PV = nRT. In this scenario, temperature (T) and moles (n) are constant, so P is inversely proportional to V. Reducing the volume increases the pressure to maintain the equation’s balance. Therefore, compression results in an increase in pressure.