Gases play a fundamental role in physical chemistry, influencing various biological and chemical processes. On the MCAT, you’ll explore gas laws, the behavior of ideal and real gases, and key concepts such as pressure, volume, temperature, and moles. Understanding kinetic molecular theory, partial pressures, and diffusion is crucial, as these concepts are essential for mastering the general chemistry and physics sections of the exam.
Learning Objectives
In studying “Gas Phase” for the MCAT, you should learn to understand the fundamental properties of gases, including pressure, temperature, volume, and amount in moles. Study the ideal gas law (PV = nRT) and how it relates to real-world gases, along with deviations from ideal behavior under non-standard conditions. Analyze gas mixtures through Dalton’s law of partial pressures and Graham’s law of diffusion and effusion. Understand kinetic molecular theory to explain gas behavior at molecular levels. Evaluate real gas behavior using the Van der Waals equation and learn how changes in temperature and pressure affect phase transitions. Additionally, apply these concepts to biological systems and chemical reactions involving gases, such as respiration and gas exchange.
Fundamental Properties of Gases
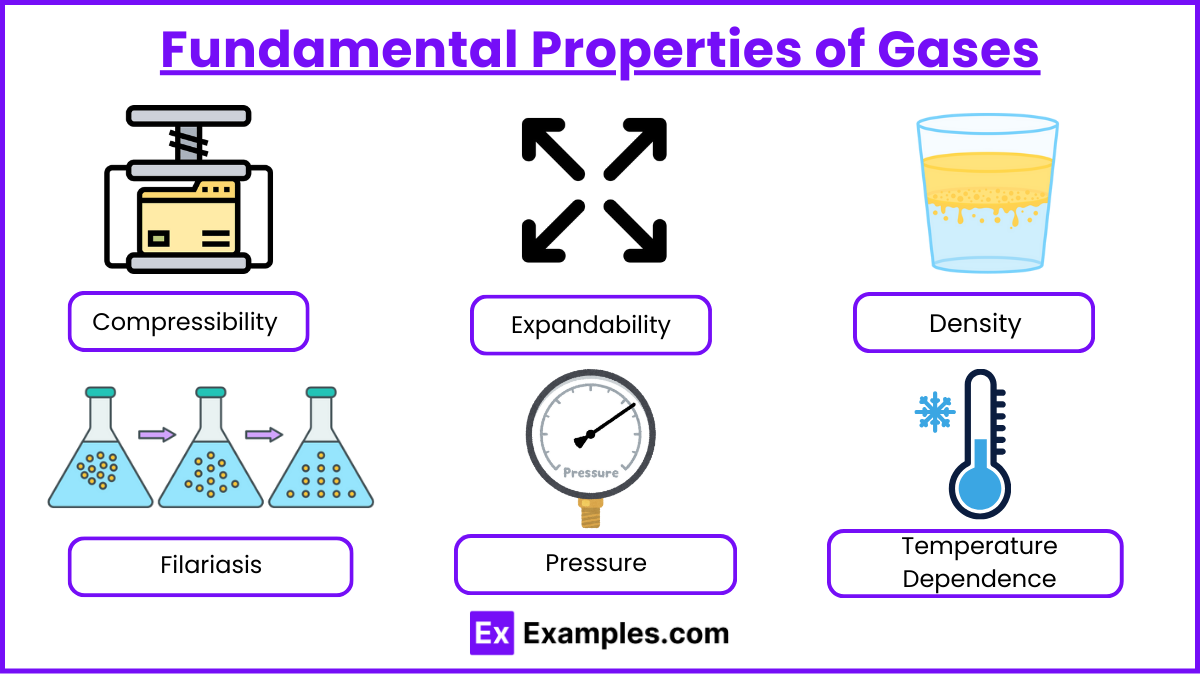
1. Compressibility
Gases are highly compressible compared to liquids and solids. Under increased pressure, the molecules of a gas can be forced closer together, significantly reducing the volume of the gas. This property is exploited in numerous applications, such as in the operation of gas compressors, refrigeration cycles, and internal combustion engines.
2. Expandability
When the pressure on a gas is reduced, it expands to fill the available space. Unlike solids and liquids, a gas will expand indefinitely in the absence of a container, spreading out evenly in all directions. This characteristic is crucial for understanding the behavior of gases in open systems and environments like the atmosphere.
3. Density
The density of a gas is generally much lower than that of liquids and solids, and it varies significantly with changes in pressure and temperature. Gas density is a critical factor in calculations involving buoyancy, diffusion, and dynamics in atmospheric phenomena.
4. Diffusivity
Gases mix with other gases spontaneously through the process of diffusion. The rate of diffusion is influenced by the temperature and the masses of the molecules involved. This property is essential in fields such as environmental science, where it helps explain the mixing of pollutants in the air.
5. Pressure
Gas molecules exert pressure on any surface with which they are in contact. This pressure is a result of the force of gas molecules colliding with surfaces. Understanding pressure is vital for everything from meteorology (e.g., weather prediction) to engineering (e.g., design of pressure vessels).
6. Temperature Dependence
The properties of gases are highly sensitive to changes in temperature. According to the ideal gas law (PV = nRT), pressure and volume are directly proportional to temperature when the number of molecules (n) and the gas constant (R) are constant. This relationship is foundational for thermodynamics and energy engineering.
Kinetic Molecular Theory of Gases
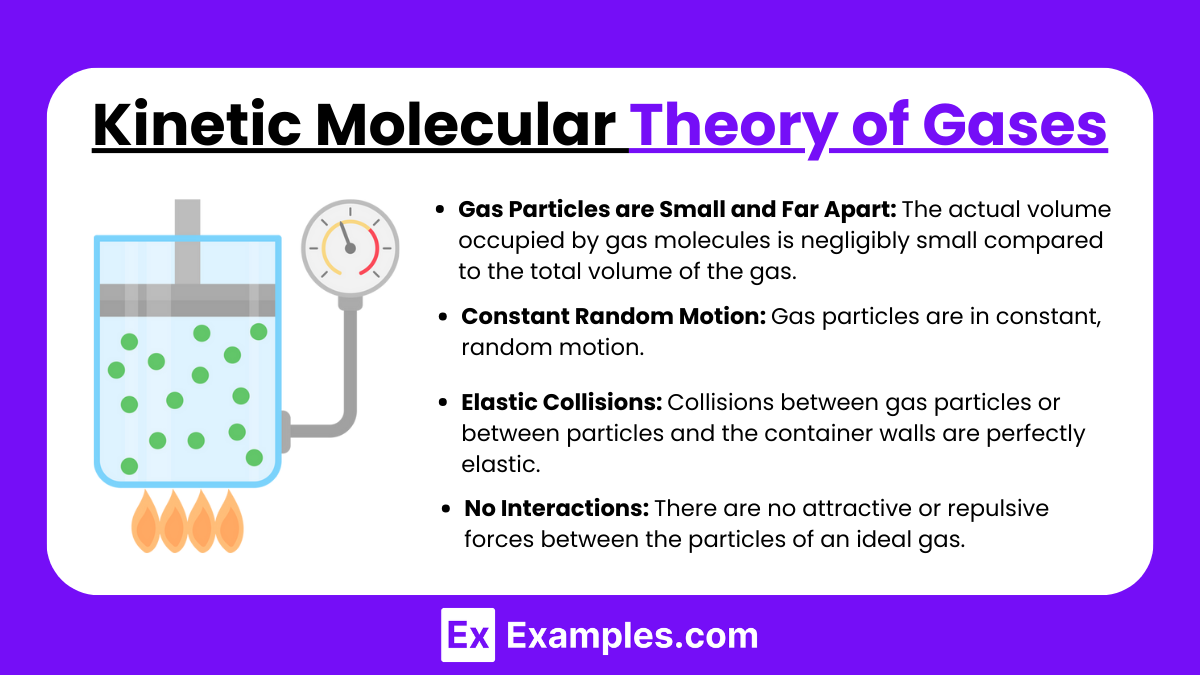
- Gas Particles are Small and Far Apart: The actual volume occupied by gas molecules is negligibly small compared to the total volume of the gas. This implies that most of the volume of a gas is empty space.
- Constant Random Motion: Gas particles are in constant, random motion. They move in straight lines until they collide with either another particle or the walls of their container.
- Elastic Collisions: Collisions between gas particles or between particles and the container walls are perfectly elastic. This means that there is no net loss of kinetic energy from these collisions.
- No Interactions: There are no attractive or repulsive forces between the particles of an ideal gas. This allows for the simplification of many calculations, though it deviates from reality where such forces can exist, particularly under high pressures and low temperatures.
- Kinetic Energy and Temperature: The average kinetic energy of gas particles is proportional to the temperature of the gas in kelvins. Higher temperatures correspond to higher average kinetic energies.
Phase Transitions and the Effect of Temperature and Pressure
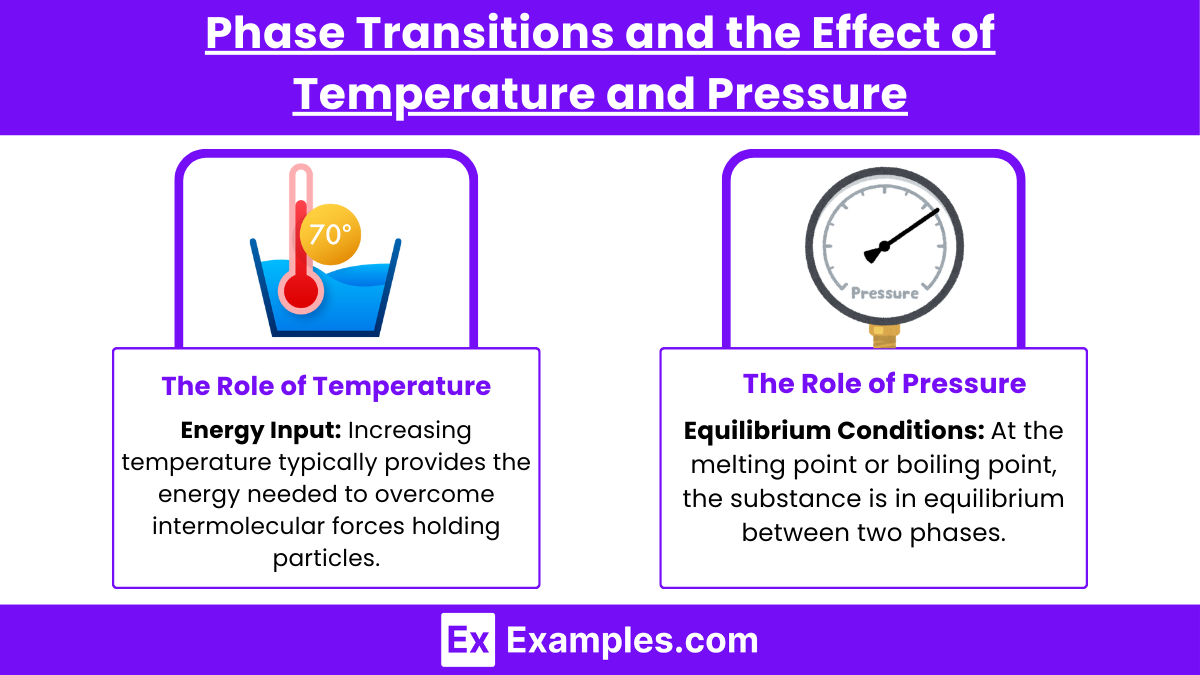
Phase transitions refer to the changes in the state of matter of a substance, typically from solid to liquid (melting), liquid to gas (vaporization), or directly from solid to gas (sublimation), among other transitions like freezing and condensation. These transformations are fundamentally influenced by changes in temperature and pressure, making an understanding of these effects essential for fields ranging from materials science to meteorology and chemical engineering.
Understanding Phase Transitions
Types of Phase Transitions:
- Melting: Transition from solid to liquid.
- Freezing: Transition from liquid to solid.
- Vaporization: Transition from liquid to gas.
- Condensation: Transition from gas to liquid.
- Sublimation: Transition from solid directly to gas.
- Deposition: Transition from gas directly to solid.
Each of these transitions occurs at specific temperature and pressure conditions that depend on the substance’s unique properties.
The Role of Temperature
Temperature is a primary driver of phase transitions:
- Energy Input: Increasing temperature typically provides the energy needed to overcome intermolecular forces holding particles together in a solid or liquid state. For example, heating ice increases the kinetic energy of its molecules enough to break the hydrogen bonds between them, leading to melting.
- Equilibrium Conditions: At the melting point or boiling point, the substance is in equilibrium between two phases (solid-liquid for melting, liquid-gas for boiling). Changes in temperature can shift this equilibrium, prompting a phase change.
The Role of Pressure
Pressure can also significantly influence phase transitions:
- Direct Influence: Increasing pressure on a substance tends to favor the denser phase. For example, increasing pressure on water tends to favor the liquid phase over the vapor phase, effectively raising its boiling point.
- Phase Diagrams: Each substance has a phase diagram that maps phases under different temperature and pressure conditions. These diagrams are crucial for understanding and predicting the conditions under which phase transitions occur.
Gas Mixtures and Dalton’s Law of Partial Pressures
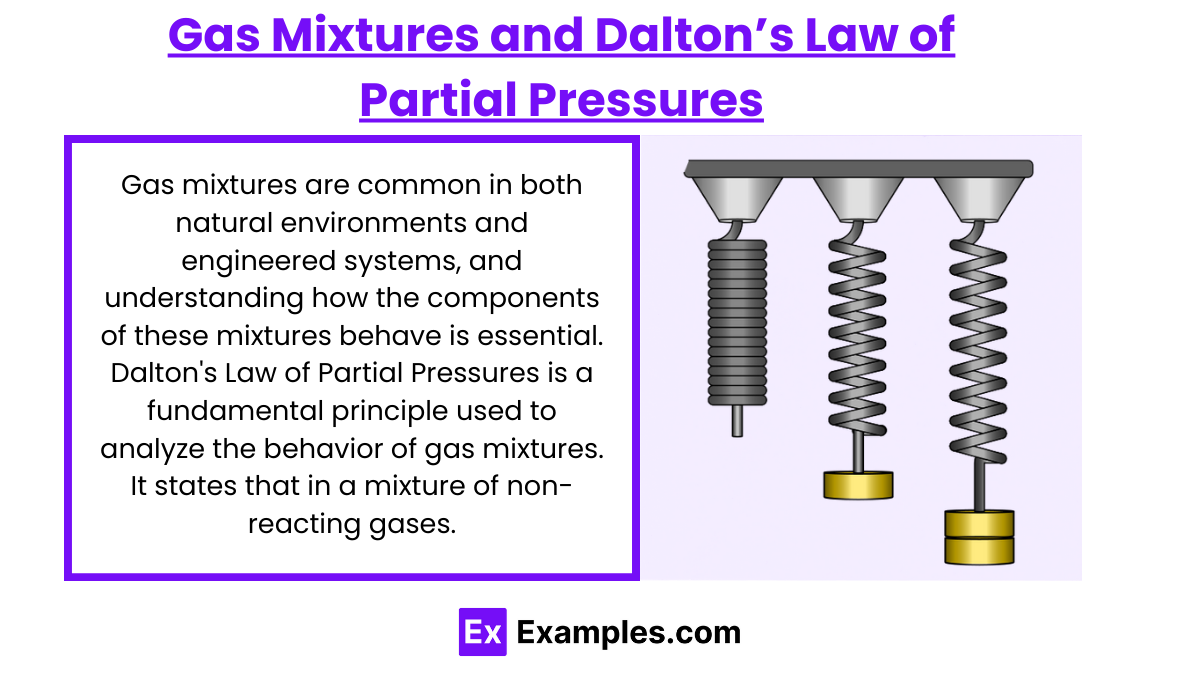
Gas mixtures are common in both natural environments and engineered systems, and understanding how the components of these mixtures behave is essential. Dalton’s Law of Partial Pressures is a fundamental principle used to analyze the behavior of gas mixtures. It states that in a mixture of non-reacting gases, the total pressure exerted is equal to the sum of the partial pressures of the individual gases.
Dalton’s Law of Partial Pressures
Definition: Dalton’s Law can be formally expressed as: Ptotal = P1+P2+P3+…+Pn Ptotal is the total pressure of the mixture, and P1, P2, P3, …, Pn are the partial pressures of each gas component in the mixture.
Partial Pressure: The partial pressure of any single gas in a mixture is the pressure that gas would exert if it alone occupied the entire volume of the mixture at the same temperature. This concept is crucial because it implies that each gas in a mixture behaves independently of the others.
Applications of Dalton’s Law
1. Atmospheric Science:
- Atmospheric Composition: Earth’s atmosphere is a mixture of nitrogen, oxygen, argon, carbon dioxide, and other gases. Dalton’s Law helps in determining the contribution of each gas to atmospheric pressure, which is important for various calculations in meteorology and climatology.
2. Scuba Diving:
- Breathing Gas Management: Scuba divers use gas mixtures such as air, nitrox, or trimix for breathing underwater. Dalton’s Law is used to calculate the partial pressures of oxygen, nitrogen, and helium to avoid oxygen toxicity and nitrogen narcosis, and to determine decompression schedules.
3. Medical Applications:
- Anesthesia: In anesthesiology, Dalton’s Law helps in determining the correct mixture of gases (such as oxygen and anesthetic gases) to ensure that patients receive the right amount of oxygen and anesthetic during surgery.
4. Chemical Engineering:
- Industrial Gas Use: In processes like gas welding, where mixtures of gases (such as acetylene and oxygen) are used, Dalton’s Law helps in setting the correct flow rates for each gas to achieve optimal combustion or desired chemical reactions.
Examples
Example 1: Air in the Atmosphere
The Earth’s atmosphere is a mixture of gases, primarily nitrogen and oxygen, along with trace amounts of other gases like carbon dioxide and argon. The behavior of air as a gas is influenced by factors such as temperature, pressure, and volume. For example, as the temperature increases, the air expands, causing the pressure to decrease if the volume remains constant, illustrating the properties of gases.
Example 2: Helium in Balloons
When helium gas is filled into a balloon, it occupies the space inside the balloon and exerts pressure on the walls. Helium is less dense than the surrounding air, allowing the balloon to float. This example demonstrates buoyancy and the principle that gases will rise when they are lighter than the fluid (air) they are in.
Example 3: Gas in a Syringe
When a syringe is filled with gas, the gas molecules are in constant motion, colliding with each other and the walls of the syringe. If the plunger is pushed down, the volume of gas decreases, and the pressure inside the syringe increases. This behavior showcases the compressibility of gases and the relationship between pressure and volume, where gases can be easily compressed compared to liquids and solids.
Example 4: Carbon Dioxide in Carbonated Beverages
In a sealed bottle of soda, carbon dioxide gas is dissolved in the liquid under high pressure. When the bottle is opened, the pressure is released, and the gas comes out of solution, forming bubbles. This example illustrates how gases can exist in equilibrium with liquids and how pressure changes can lead to phase transitions, such as gas escaping from a solution.
Example 5: Exhaled Air
When humans exhale, the air released is primarily composed of nitrogen, oxygen, and carbon dioxide. The process of breathing demonstrates the gas phase in a biological context, where the exchange of gases occurs in the lungs. The gas molecules diffuse from areas of higher concentration (in the blood) to lower concentration (in the lungs), exemplifying the principles of gas diffusion and partial pressure.
Practice Questions
Question 1
Which of the following statements is true regarding gases compared to liquids and solids?
A) Gases have a definite shape and volume.
B) Gases are incompressible and do not expand to fill their containers.
C) Gases have particles that are widely spaced and move freely.
D) Gases cannot flow and are rigid.
Correct Answer: C) Gases have particles that are widely spaced and move freely.
Explanation: Gases are characterized by having particles that are far apart, allowing them to move freely and fill the volume of their containers. Unlike solids, which have a definite shape and volume, and liquids, which have a definite volume but take the shape of their container, gases do not have a fixed shape or volume. They can be compressed and expand to fill any space, making option C the correct statement.
Question 2
What occurs to a gas when it is heated in a closed container?
A) The gas contracts and decreases in pressure.
B) The gas expands and increases in pressure.
C) The gas remains at the same volume and pressure.
D) The gas turns into a liquid.
Correct Answer: B) The gas expands and increases in pressure.
Explanation: When a gas is heated in a closed container, the increase in temperature causes the gas particles to gain kinetic energy, which leads to more frequent and forceful collisions with the walls of the container. As a result, the pressure of the gas increases. If the container were able to expand, the gas would also expand. However, in a closed container, the increase in temperature directly translates into an increase in pressure, making option B the correct answer.
Question 3
Which of the following best describes the behavior of gas molecules in a confined space?
A) They vibrate in place and do not change position.
B) They remain stationary and do not interact with each other.
C) They move rapidly and collide with each other and the walls of the container.
D) They form fixed patterns and maintain specific distances from each other.
Correct Answer: C) They move rapidly and collide with each other and the walls of the container.
Explanation: Gas molecules are in constant motion, moving rapidly and colliding with one another and the walls of their container. This kinetic activity is what allows gases to fill the space available to them. Unlike solids, which vibrate in fixed positions, or liquids, which have less freedom of movement, gas molecules exhibit random motion and occupy the entire volume of their container. Therefore, option C accurately describes the behavior of gas molecules.