Gene control involves the regulation of gene expression to ensure proper cellular function and organismal development. This process includes various mechanisms like transcription factors, chromatin remodeling, and RNA processing. By managing when, where, and how much genes are expressed, cells can respond to environmental changes and maintain homeostasis, which is essential for understanding complex biological systems on the MCAT.
Learning Objectives
In studying “Gene Control” for the MCAT, you should learn to understand the mechanisms by which gene expression is regulated in prokaryotic and eukaryotic cells. Analyze processes such as transcriptional regulation, epigenetic modifications (DNA methylation, histone modification), and the role of transcription factors. Evaluate how operons, enhancers, silencers, and non-coding RNAs contribute to gene regulation. Additionally, explore the implications of gene control in cell differentiation, development, and disease. Apply these concepts to MCAT practice passages, interpreting data related to gene expression and regulatory mechanisms.
1. Gene Expression and Regulation
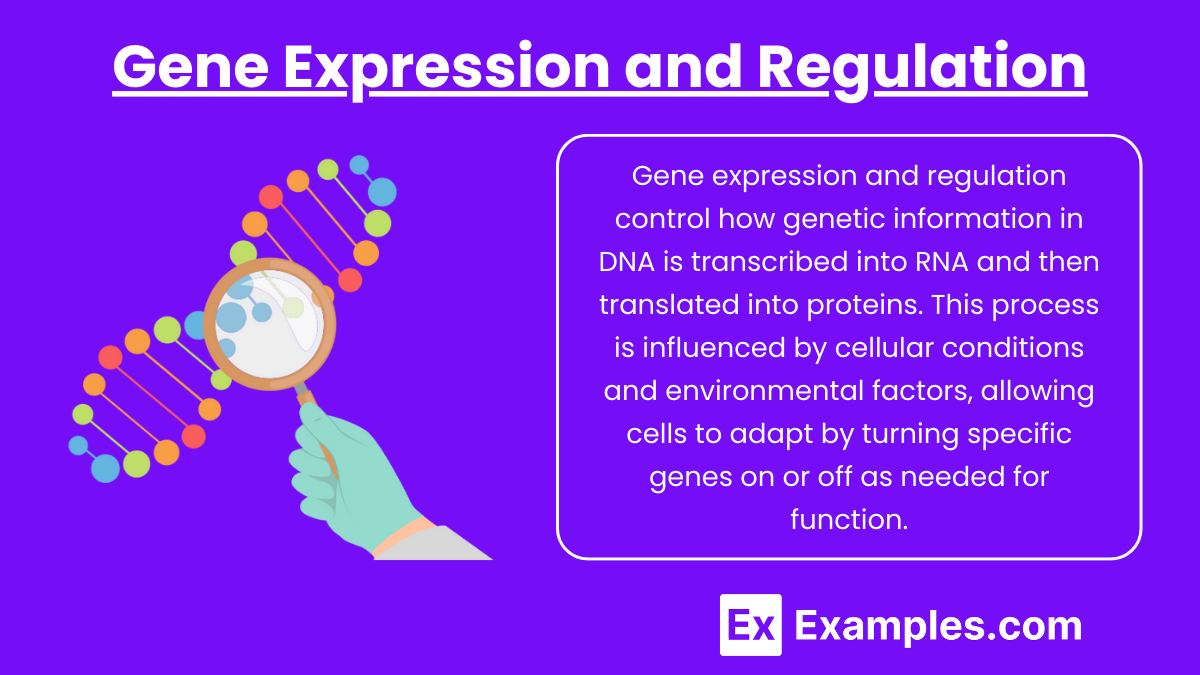
- Overview: Gene expression refers to the process by which the genetic information encoded in DNA is used to synthesize functional products, typically proteins. This involves two main stages: transcription (DNA to RNA) and translation (RNA to protein).
- Importance of Regulation: Cells regulate gene expression to adapt to environmental changes, conserve energy, and ensure that proteins are synthesized only when needed. Regulation occurs at multiple stages: transcriptional (whether mRNA is produced), post-transcriptional (mRNA processing), translational (mRNA to protein), and post-translational (protein modifications).
- Regulatory Elements: DNA sequences such as promoters, enhancers, silencers, and response elements are critical for controlling gene expression. Promoters are binding sites for RNA polymerase, while enhancers and silencers can increase or decrease transcription rates, often by binding transcription factors.
2. Prokaryotic Gene Control
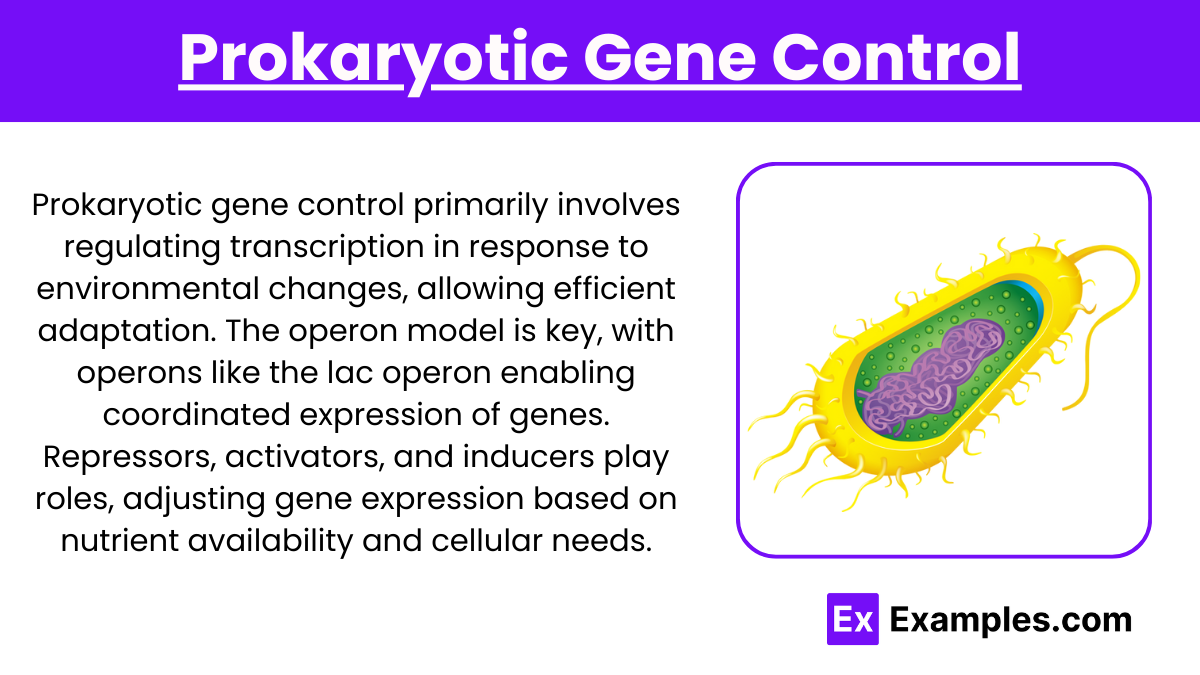
- Operons: In prokaryotes, operons are groups of genes regulated together, allowing bacteria to respond rapidly to environmental changes. Operons include structural genes, an operator, a promoter, and regulatory genes.
- Lac Operon: An example of an inducible system, the lac operon controls the breakdown of lactose. When lactose is present, it binds to the repressor protein, allowing transcription of genes needed to metabolize lactose.
- Trp Operon: An example of a repressible system, the trp operon regulates the production of tryptophan. When tryptophan is abundant, it binds to the repressor, enabling it to block transcription and conserve resources.
- Regulatory Proteins:
- Repressors: Bind to the operator to block RNA polymerase, stopping transcription. In inducible operons, the presence of a specific molecule (e.g., lactose) can remove the repressor.
- Activators: Enhance the binding of RNA polymerase to the promoter. In the absence of glucose, the lac operon is activated by cAMP, which helps recruit RNA polymerase.
3. Eukaryotic Gene Control
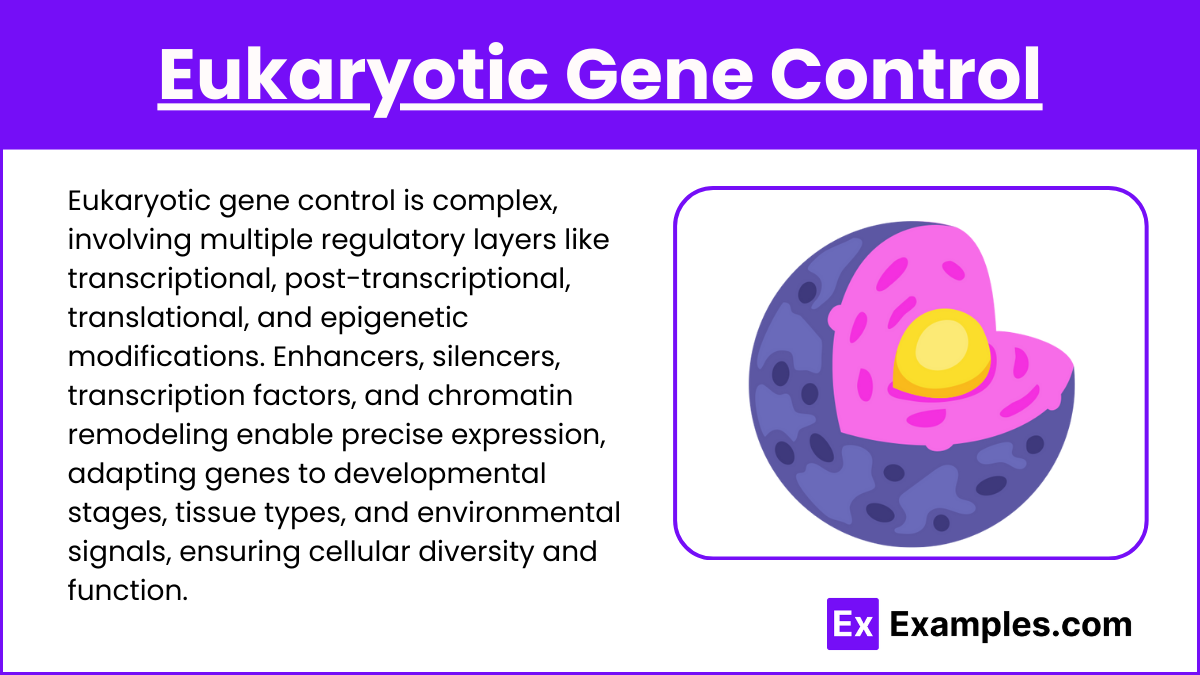
- Chromatin Structure and Remodeling: DNA is wrapped around histone proteins to form nucleosomes, which can be tightly packed (heterochromatin) or loosely packed (euchromatin).
- Histone Modification: Acetylation of histones by acetyltransferases often loosens chromatin, allowing gene expression, while deacetylation condenses chromatin, repressing gene expression. Methylation of histones can either activate or repress genes depending on the specific amino acids modified.
- Transcription Factors: In eukaryotes, transcription factors (TFs) are proteins that bind to specific DNA sequences, enabling or inhibiting the recruitment of RNA polymerase.
- Enhancers and Silencers: Enhancers are DNA sequences that increase transcription when bound by activator TFs. Silencers bind repressors to decrease transcription.
- RNA Processing and Splicing: After transcription, eukaryotic pre-mRNA undergoes modifications:
- 5′ Capping: Adds a modified guanine cap to the mRNA’s 5′ end, crucial for mRNA stability and initiation of translation.
- Polyadenylation: The addition of a poly-A tail to the 3′ end, enhancing stability and export from the nucleus.
- Alternative Splicing: Allows a single gene to code for multiple proteins by varying exon inclusion, which increases protein diversity.
- Non-Coding RNAs: miRNAs and siRNAs are small RNAs that regulate gene expression post-transcriptionally. They can degrade target mRNA or inhibit translation, influencing developmental processes and cell differentiation.
4. Epigenetic Regulation
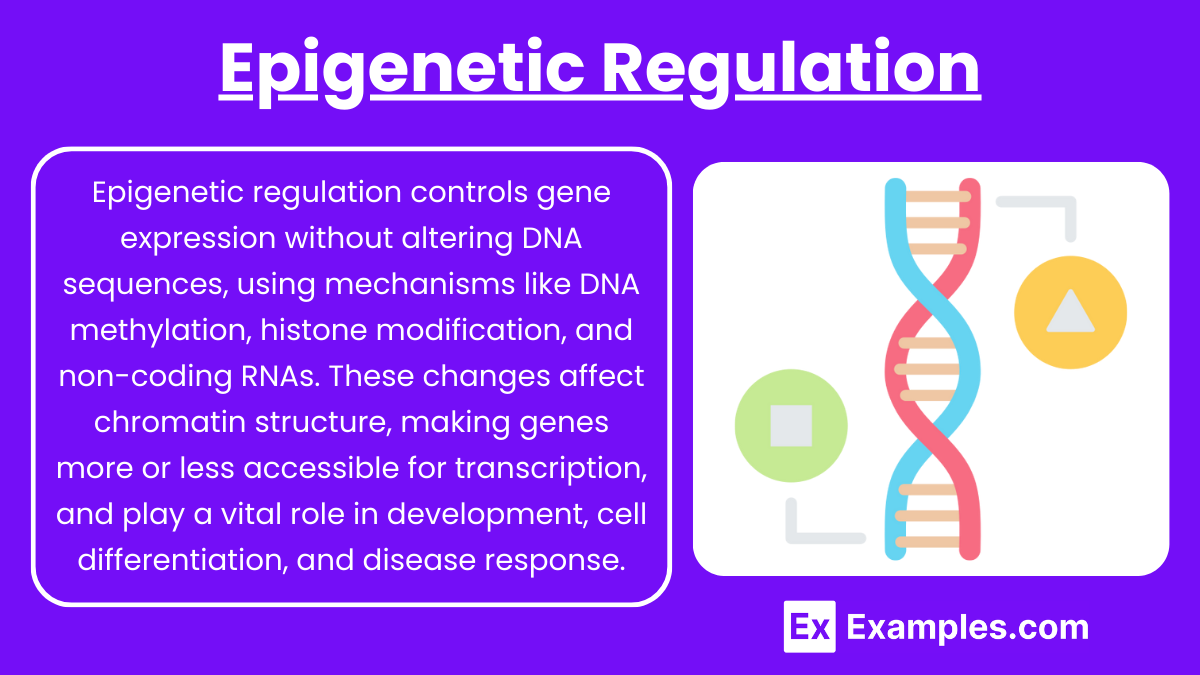
- DNA Methylation: Methyl groups added to cytosine residues in CpG islands can silence genes by preventing transcription factors from accessing DNA. This is vital for processes such as X-inactivation (silencing one X chromosome in female mammals) and genomic imprinting (differential expression of parental alleles).
- Histone Code: Histone modifications (e.g., acetylation, methylation) collectively make up the “histone code,” which influences chromatin structure and gene accessibility. This code is dynamic and can change in response to environmental factors.
- Long-Term Effects: Epigenetic changes can be passed to daughter cells during cell division, affecting gene expression across generations without altering DNA sequence.
5. Gene Control in Cancer and Disease
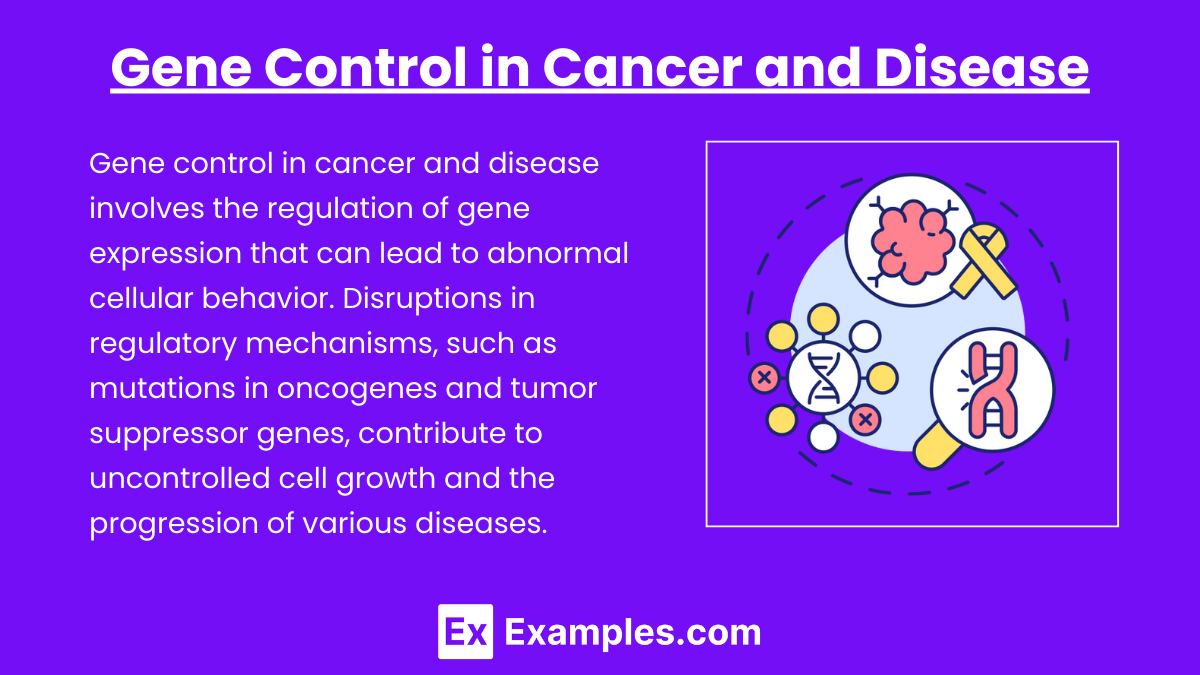
- Tumor Suppressors and Oncogenes: Mutations in these genes can lead to uncontrolled cell growth.
- Tumor Suppressors: Genes like p53 act as brakes on the cell cycle. When mutated, they can lead to unchecked cell division.
- Oncogenes: Mutated or overexpressed versions of normal genes (proto-oncogenes) that drive cell growth. Examples include Ras and Myc.
- Gene Therapy: Understanding gene regulation allows for targeted therapies. Gene therapy strategies can involve correcting defective genes, silencing harmful ones, or introducing new genes.
Examples
Example 1: Point Mutation
A single nucleotide change, as in sickle cell anemia, where a substitution in the hemoglobin gene alters protein structure, affecting oxygen transport and causing red blood cells to become crescent-shaped.
Example 2: Frameshift Mutation
Insertions or deletions of nucleotides that disrupt the reading frame, as seen in cystic fibrosis, where a deletion in the CFTR gene results in defective chloride transport, leading to thick mucus buildup.
Example 3: Nonsense Mutation
A point mutation converts a codon to a stop codon, prematurely ending protein synthesis. In Duchenne muscular dystrophy, this truncation disrupts dystrophin protein production, weakening muscle integrity.
Example 4: Missense Mutatio
A nucleotide substitution changes one amino acid in a protein. Marfan syndrome results from a missense mutation in the FBN1 gene, affecting connective tissue and causing skeletal, ocular, and cardiovascular abnormalities.
Example 5: Silent Mutation
A mutation alters a codon without changing the amino acid due to genetic code redundancy. While often benign, some silent mutations can influence gene regulation or splicing, impacting protein function indirectly.
Practice Questions
Question 1:
Which type of mutation results in a premature stop codon, potentially truncating the protein?
A) Silent
B) Missense
C) Nonsense
D) Frameshift
Answer: C) Nonsense
Explanation: Nonsense mutations introduce a stop codon, halting translation prematurely and often leading to a nonfunctional, truncated protein.*
Question 2:
A deletion of two nucleotides likely results in which type of mutation?
A) Silent
B) Frameshift
C) Missense
D) Nonsense
Answer: B) Frameshift
Explanation: Deleting two nucleotides disrupts the reading frame, causing a frameshift, which alters subsequent amino acids and significantly impacts protein function.*
Question 3:
In which mutation does a single nucleotide change, but the encoded amino acid remains the same?
A) Silent
B) Nonsense
C) Missense
D) Frameshift
Answer: A) Silent
Explanation: Silent mutations change one nucleotide without altering the amino acid, thanks to the redundancy in the genetic code, often leaving protein function unchanged.