Infrared and Ultraviolet/Visible spectroscopy are key techniques in MCAT chemistry. Infrared spectroscopy identifies molecular vibrations and functional groups, while UV/Vis spectroscopy detects electronic transitions, revealing information about conjugated systems and chromophores. Both techniques help analyze chemical structures and molecular interactions efficiently.
Learning Objectives
In studying “Infrared (IR) and Ultraviolet/Visible (UV-Vis) Spectroscopy” for the MCAT, you should learn to understand the principles behind how molecules absorb infrared and ultraviolet/visible light. Identify functional groups using IR spectra based on their characteristic absorption bands. Understand the correlation between molecular vibrations and IR absorption, including stretching and bending modes. Recognize the role of conjugation and electronic transitions in UV-Vis spectroscopy, and interpret how changes in wavelength (λmax) relate to molecular structure. Evaluate applications of these spectroscopic techniques in identifying organic compounds and tracking chemical reactions. Additionally, understand how these techniques aid in characterizing molecules relevant to biochemistry and medicine.
Principles of Infrared (IR) and Ultraviolet/Visible (UV-Vis) Spectroscopy
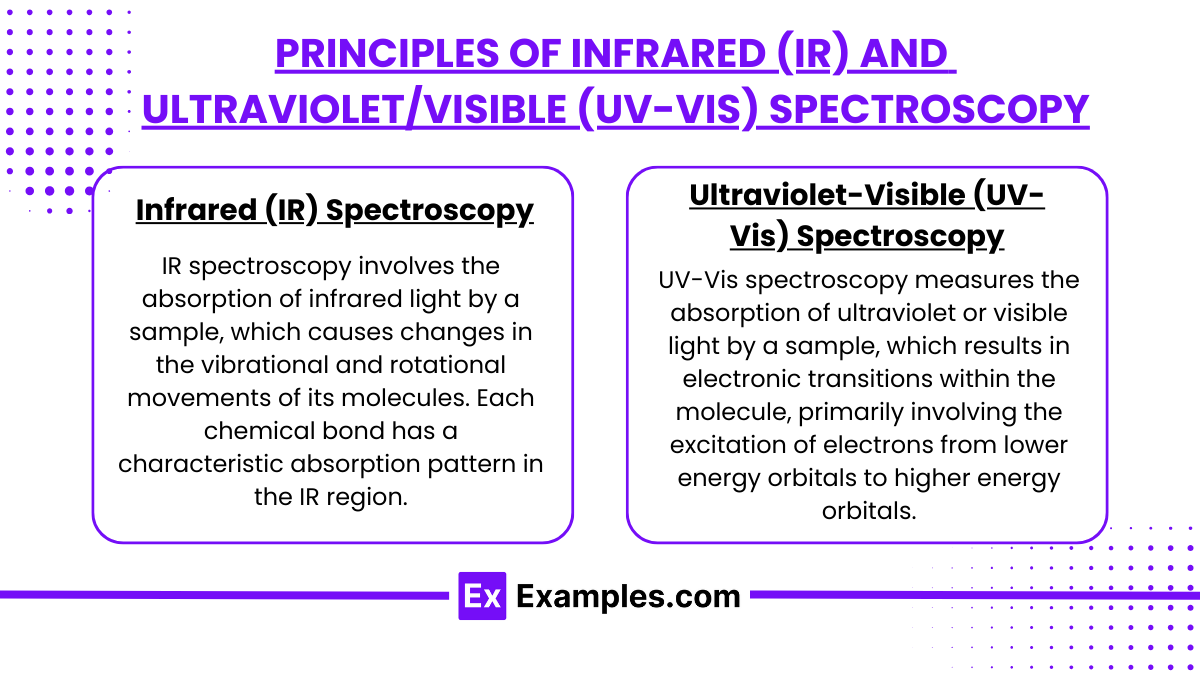
Infrared (IR) and ultraviolet-visible (UV-Vis) spectroscopy are two fundamental analytical techniques used extensively in chemistry, biochemistry, and materials science to determine the composition and properties of substances. Both methods exploit the interaction of light with matter but operate in different regions of the electromagnetic spectrum, each providing unique insights into molecular structure and dynamics.
Infrared (IR) Spectroscopy
IR spectroscopy involves the absorption of infrared light by a sample, which causes changes in the vibrational and rotational movements of its molecules. Each chemical bond has a characteristic absorption pattern in the IR region, which can be used to identify functional groups and molecular structures.
Key Principles:
- Vibrational Transitions: IR spectroscopy is primarily concerned with the vibrational transitions of molecules. When IR radiation is absorbed, molecules are excited from a lower to a higher vibrational state. The energy required for these transitions corresponds to the IR region of the electromagnetic spectrum.
- Fingerprint Region: The region from 4000 cm⁻¹ to 400 cm⁻¹ in the IR spectrum is known as the fingerprint region. Each organic compound has a unique absorption pattern in this region, which can be used like a fingerprint to identify the compound.
- Instrumentation: An IR spectrometer typically consists of an IR light source, a sample holder, a detector, and a processor to record and display data. The sample can be analyzed in various forms, including gases, liquids, and solids.
Applications:
- Functional Group Identification: Helps in identifying and characterizing functional groups in organic and inorganic molecules.
- Material Science: Used in polymer testing and identification, monitoring of formulations, and determination of molecular orientation.
Ultraviolet-Visible (UV-Vis) Spectroscopy
UV-Vis spectroscopy measures the absorption of ultraviolet or visible light by a sample, which results in electronic transitions within the molecule, primarily involving the excitation of electrons from lower energy orbitals to higher energy orbitals.
Key Principles:
- Electronic Transitions: UV-Vis spectroscopy is based on electronic transitions, specifically the promotion of electrons to higher energy states upon absorbing light at specific wavelengths.
- Beer-Lambert Law: This law relates the absorption of light to the properties of the material through which the light is traveling. It is given by A= ϵ⋅c⋅l\, where A is the absorbance, ϵ is the molar absorptivity, c is the concentration of the solution, and lll is the path length through the sample.
- Instrumentation: A typical UV – Vis spectrometer includes a light source emitting UV and visible light, a monochromator to select specific wavelengths, a sample compartment, and a detector to measure the intensity of transmitted light.
Applications:
- Concentration Determination: Widely used in biochemistry to determine the concentration of proteins, nucleic acids, and other biomolecules.
- Chemical Analysis: Helps in identifying and characterizing compounds, especially in complex mixtures.
Identifying Functional Groups Using IR Spectra
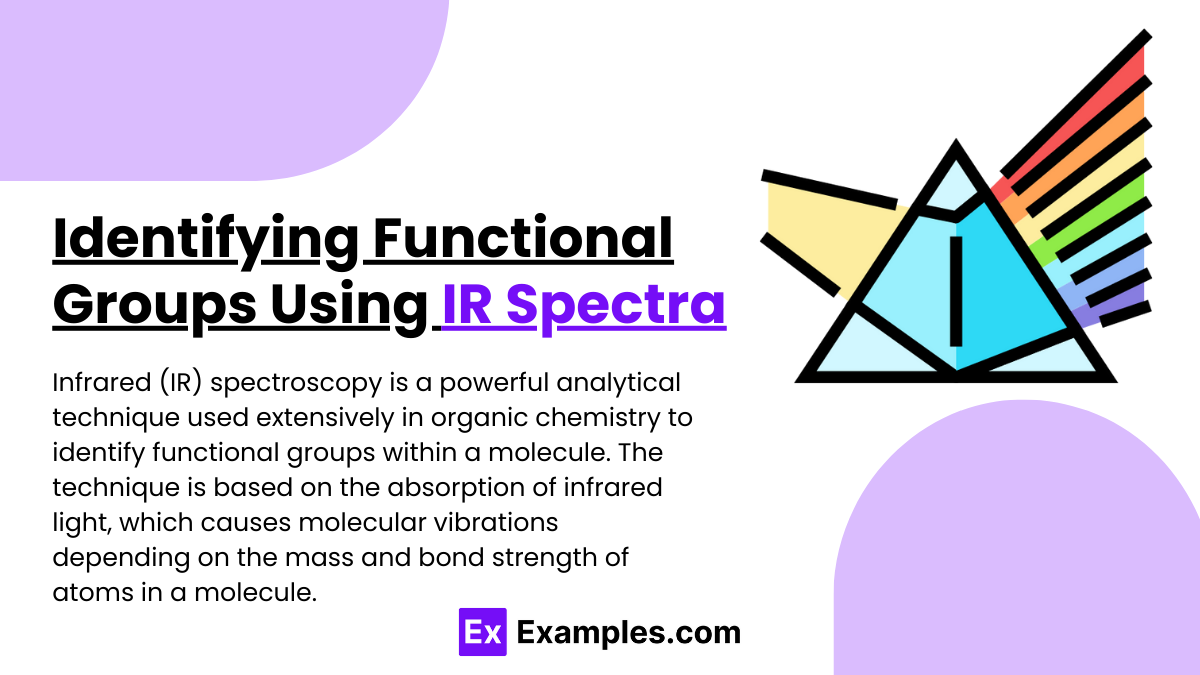
Infrared (IR) spectroscopy is a powerful analytical technique used extensively in organic chemistry to identify functional groups within a molecule. The technique is based on the absorption of infrared light, which causes molecular vibrations depending on the mass and bond strength of atoms in a molecule. Each functional group has characteristic vibration frequencies that are often unique and identifiable on an IR spectrum.
How IR Spectra Identify Functional Groups
- Characteristic Absorption Bands:
- Every functional group in an organic compound absorbs IR radiation at specific frequencies, creating a unique pattern or signature. These absorption bands correspond to different vibrational modes such as stretching, bending, or twisting.
- Region of the Spectrum:
- The IR spectrum is generally divided into two regions:
- Functional Group Region (4000-1500 cm⁻¹): This area contains most of the sharp, distinct peaks that are diagnostic of particular functional groups.
- Fingerprint Region (1500-400 cm⁻¹): This region is more complex and is specific to individual molecules. It is often used to distinguish between two similar compounds.
- The IR spectrum is generally divided into two regions:
Common Functional Groups and Their IR Absorption Ranges
- Hydroxyl Group (OH):
- Bond Type: Stretching
- Frequency Range: 3200-3600 cm⁻¹ (broad, often with a rounded peak due to hydrogen bonding)
- Found in: Alcohols, phenols, carboxylic acids
- Carbonyl Group (C=O):
- Bond Type: Stretching
- Frequency Range: 1700-1750 cm⁻¹ (sharp, strong peak)
- Found in: Ketones, aldehydes, carboxylic acids, esters, amides
- Amine Group (NH):
- Bond Type: Stretching
- Frequency Range:
- Primary amines: 3300-3500 cm⁻¹ (two bands, medium)
- Secondary amines: 3300-3500 cm⁻¹ (one band, medium)
- Found in: Amines
- Alkene (C=C):
- Bond Type: Stretching
- Frequency Range: 1620-1680 cm⁻¹ (moderate intensity)
- Found in: Alkenes
- Nitrile Group (C≡N):
- Bond Type: Stretching
- Frequency Range: 2210-2260 cm⁻¹ (sharp, medium intensity)
- Found in: Nitriles
- Alkyne Group (C≡C):
- Bond Type: Stretching
- Frequency Range: 2100-2260 cm⁻¹ (moderate intensity)
- Found in: Alkynes (frequency varies slightly depending on substitution)
Using IR Spectroscopy
- Sample Preparation: Samples can be prepared in several forms, including neat (pure substance), solution, mulls (ground with mineral oil), or films.
- Interpretation: Analysts compare the absorption peaks in the sample’s IR spectrum with known values from IR correlation charts or databases. Key factors include peak position, shape, and intensity.
- Confirmation: Due to the complexity of some spectra, particularly in molecules with multiple functional groups, other analytical techniques like NMR (Nuclear Magnetic Resonance) spectroscopy or mass spectrometry may be used alongside IR spectroscopy for confirmation.
Molecular Vibrations and IR Absorption: Stretching and Bending Modes
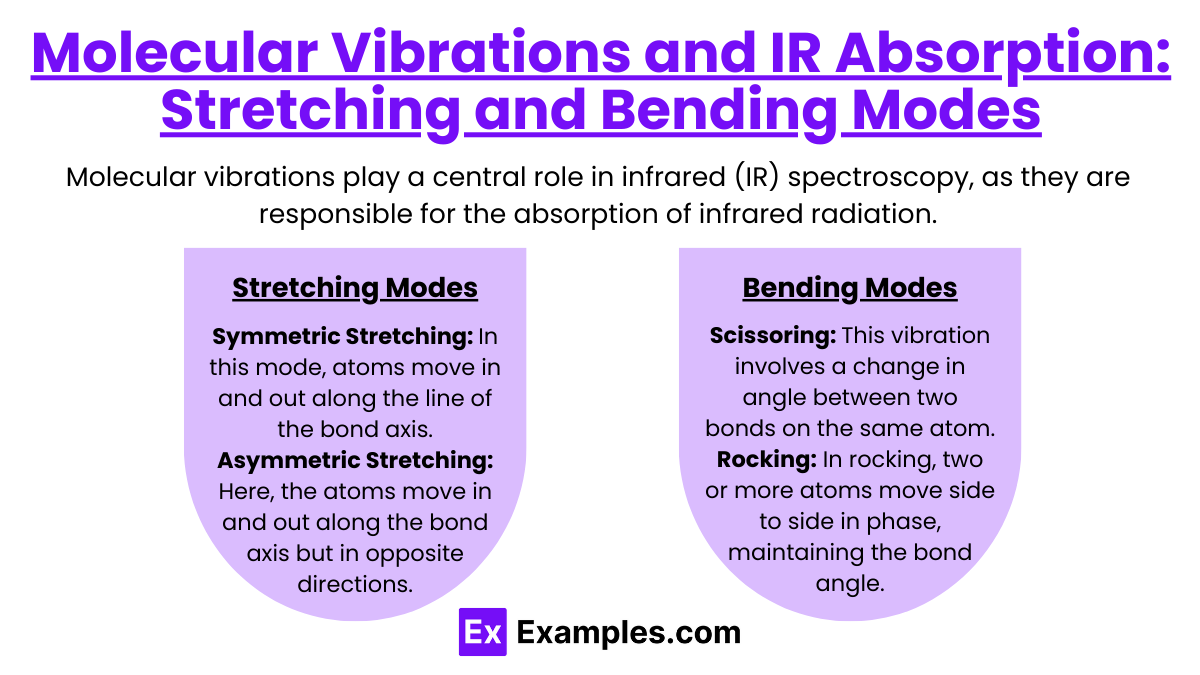
Molecular vibrations play a central role in infrared (IR) spectroscopy, as they are responsible for the absorption of infrared radiation. Every molecule with covalent bonds can absorb infrared light at frequencies that correspond to the vibrations of its atoms. These vibrations come in two primary types: stretching and bending modes. Each type gives specific insights into the molecule’s structure and helps in identifying functional groups and bond types within the molecule.
Stretching Modes
Stretching vibrations involve changes in the length of the bond between atoms. There are two types of stretching vibrations:
- Symmetric Stretching: In this mode, atoms move in and out along the line of the bond axis, maintaining symmetry about the bond axis. For example, in a molecule like carbon dioxide (CO2), the two oxygen atoms can move closer to and farther from the carbon atom simultaneously in a symmetrical fashion.
- Asymmetric Stretching: Here, the atoms move in and out along the bond axis but in opposite directions, breaking symmetry about the bond axis. In the case of CO2, while one oxygen atom moves toward the carbon, the other moves away, creating an asymmetric motion.
Stretching vibrations generally occur at higher frequencies than bending vibrations and are often easier to identify in an IR spectrum due to their relatively strong absorption peaks.
Bending Modes
Bending vibrations involve changes in the angle between bonds connecting three or more atoms. There are several types of bending vibrations:
- Scissoring: This vibration involves a change in angle between two bonds on the same atom, resembling the motion of a pair of scissors. The bonds move toward or away from each other but remain in the same plane.
- Rocking: In rocking, two or more atoms move side to side in phase, maintaining the bond angle but shifting the position of the whole group sideways.
- Wagging: This involves moving two or more atoms up and down out of the plane of the molecule, resembling a wagging motion.
- Twisting: Similar to wagging, twisting involves a rotation of one part of the molecule relative to another around the bond axis, moving atoms out of the plane.
Bending modes generally occur at lower frequencies than stretching modes and result in less intense IR absorption peaks.
Role of Conjugation and Electronic Transitions in UV-Vis Spectroscopy
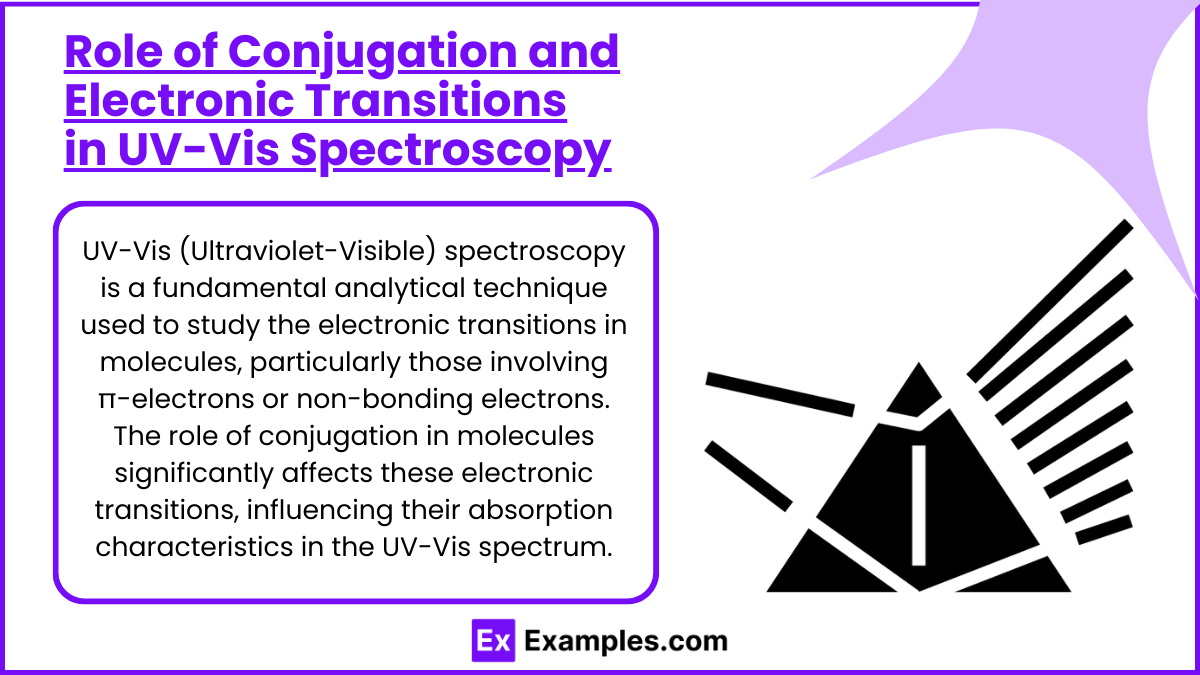
UV-Vis (Ultraviolet-Visible) spectroscopy is a fundamental analytical technique used to study the electronic transitions in molecules, particularly those involving π-electrons or non-bonding electrons. The role of conjugation in molecules significantly affects these electronic transitions, influencing their absorption characteristics in the UV-Vis spectrum.
Electronic Transitions in UV-Vis Spectroscopy
Electronic transitions refer to the movement of electrons between different energy levels within a molecule upon absorption of light. In UV-Vis spectroscopy, these transitions typically involve:
- π to π* Transitions: These occur in molecules with conjugated double bonds where electrons in π (pi) orbitals are excited to π* (pi-star) antibonding orbitals. These transitions are responsible for absorption in the UV region and are highly influenced by the extent of conjugation in the molecule.
- n to σ* and n to π* Transitions: These involve non-bonding electrons (n) being excited to either σ* (sigma-star) antibonding or π* orbitals. These electrons are usually from lone pairs on atoms like oxygen or nitrogen. n to π* transitions are commonly observed in molecules containing carbonyl groups (C=O).
- σ to σ* Transitions: These involve the excitation of electrons in σ (sigma) bonding orbitals to σ* antibonding orbitals. These transitions require a high energy and typically occur at shorter wavelengths (deep UV), outside the range of most routine UV-Vis spectrophotometers.
Role of Conjugation
Conjugation involves alternating double and single bonds, which allows π-electrons to delocalize over a larger portion of the molecule. This delocalization has significant effects on the electronic structure and optical properties of the molecule:
- Lowering of Energy Gaps: Conjugation reduces the energy gap between the highest occupied molecular orbital (HOMO) and the lowest unoccupied molecular orbital (LUMO). This reduction makes it easier for electrons to transition to higher energy levels, thus requiring photons with lower energy (longer wavelengths). As a result, the absorption maximum (λmax) shifts to longer wavelengths—a phenomenon known as bathochromic or red shift.
- Intensity of Absorption: The extent of conjugation also affects the molar absorptivity (ε), a measure of how strongly a molecule absorbs light at a given wavelength. Greater delocalization typically enhances the intensity of absorption, leading to higher ε values.
Examples
Example 1: Chemical Analysis in Laboratories
Infrared (IR) spectroscopy is widely used in laboratories to identify and analyze chemical compounds. By measuring the absorption of infrared light by a sample, scientists can determine the molecular structure and functional groups present in the compound. For example, IR spectroscopy can distinguish between alcohols, ketones, and carboxylic acids based on their unique absorption patterns.
Example 2: Characterization of Polymers
Ultraviolet-visible (UV-Vis) spectroscopy is commonly employed to study the properties of polymers. This technique allows researchers to analyze the electronic transitions in polymer materials. By measuring how much UV or visible light a polymer absorbs, scientists can infer information about its conjugation length and overall molecular structure, which is critical for applications in materials science.
Example 3: Environmental Monitoring
Both IR and UV-Vis spectroscopy play a significant role in environmental monitoring. For instance, IR spectroscopy can be used to detect greenhouse gases, such as carbon dioxide and methane, in the atmosphere. By analyzing the absorption spectra of these gases, scientists can assess their concentrations and understand their impact on climate change.
Example 4: Biomedical Applications
Infrared and UV-Vis spectroscopy are essential tools in the biomedical field for diagnosing diseases and studying biological samples. For example, IR spectroscopy can be utilized to analyze tissue samples, helping to identify pathological changes associated with diseases like cancer. Similarly, UV-Vis spectroscopy is used to measure the concentration of biomolecules, such as proteins and nucleic acids, in various samples, aiding in research and diagnostic applications.
Example 5: Food Quality Assessment
Infrared and UV-Vis spectroscopy are applied in the food industry to assess the quality and safety of food products. For example, IR spectroscopy can be used to measure the moisture content and fat levels in food products, ensuring they meet quality standards. UV-Vis spectroscopy is often employed to analyze color and chemical composition, such as detecting additives or contaminants, ensuring the safety and quality of food before it reaches consumers.
Practice Questions
Question 1
What is the primary purpose of infrared (IR) spectroscopy?
A) To measure the concentration of a solution
B) To identify molecular structures and functional groups
C) To assess the color of a substance
D) To determine the physical state of a compound
Correct Answer: B) To identify molecular structures and functional groups.
Explanation: Infrared spectroscopy is primarily used to identify and analyze the molecular structures and functional groups present in a compound by measuring the absorption of infrared light. Different functional groups absorb characteristic wavelengths of infrared light, allowing scientists to deduce the types of bonds and molecular structure present in the sample. Options A, C, and D describe other analytical techniques that do not accurately represent the main function of IR spectroscopy.
Question 2
In ultraviolet-visible (UV-Vis) spectroscopy, what does the absorption of light by a sample indicate?
A) The sample’s temperature
B) The sample’s molecular weight
C) The electronic transitions of electrons within the sample
D) The physical appearance of the sample
Correct Answer: C) The electronic transitions of electrons within the sample.
Explanation: UV-Vis spectroscopy measures the absorption of ultraviolet and visible light by a sample, which corresponds to electronic transitions of electrons from lower to higher energy levels. When a sample absorbs light, it indicates that electrons are being excited to higher energy states, providing information about the sample’s electronic structure. Options A, B, and D do not relate to the fundamental principles of UV-Vis spectroscopy.
Question 3
Which of the following applications is NOT typically associated with infrared spectroscopy?
A) Identifying functional groups in organic compounds
B) Measuring the concentration of colored solutions
C) Analyzing biological tissues for disease diagnosis
D) Detecting greenhouse gases in the atmosphere
Correct Answer: B) Measuring the concentration of colored solutions.
Explanation: Infrared spectroscopy is primarily used to identify functional groups, analyze biological tissues, and detect gases, particularly by measuring their characteristic absorption of infrared light. However, measuring the concentration of colored solutions is typically done using UV-Vis spectroscopy, which analyzes the absorption of light in the visible spectrum, where color plays a significant role. Therefore, option B is the correct answer as it does not align with the typical applications of infrared spectroscopy.