Preparing for the MCAT requires a comprehensive understanding of the Krebs (Citric Acid) Cycle and oxidative phosphorylation, two key processes in cellular respiration. The Krebs Cycle, occurring in the mitochondrial matrix, oxidizes acetyl-CoA to produce NADH, FADH2, and GTP/ATP, integrating carbohydrates, fats, and proteins into metabolism. These products fuel oxidative phosphorylation in the inner mitochondrial membrane, where the electron transport chain and ATP synthase generate ATP through chemiosmosis. Mastering these interconnected processes is vital for the MCAT, as they are central to energy production, cellular regulation, and metabolic balance.
Learning Objectives
When studying the Krebs (Citric Acid) Cycle and oxidative phosphorylation for the MCAT, focus on understanding their roles in cellular respiration and energy production. Learn the sequence of reactions within the Krebs Cycle, emphasizing key enzymes, substrates, and products like NADH, FADH2, and GTP/ATP. Grasp the regulation of the cycle by feedback inhibition and its integration with other metabolic pathways, such as glycolysis and beta-oxidation. For oxidative phosphorylation, comprehend the structure and function of the electron transport chain, the role of oxygen as the terminal electron acceptor, and the mechanism of ATP synthesis through chemiosmosis. Develop the ability to connect these processes to overall metabolic homeostasis, energy yield, and their regulation under aerobic conditions, all of which are critical for MCAT biochemistry questions.
1. Krebs Cycle (Citric Acid Cycle) Overview
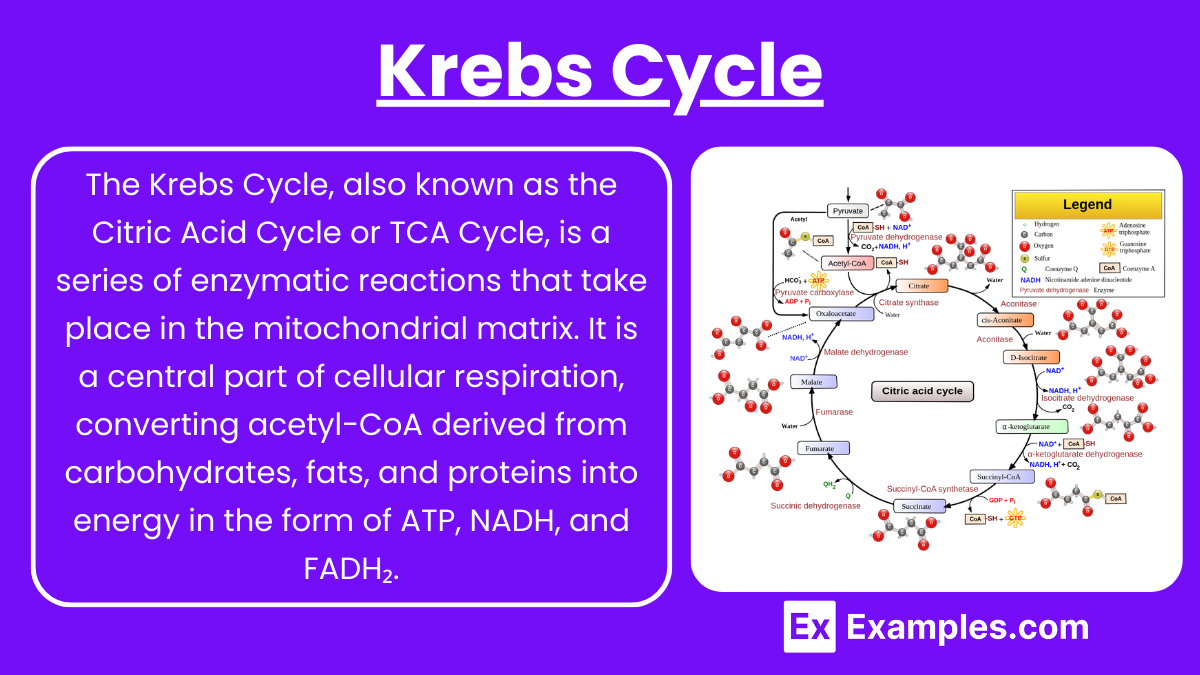
The Krebs Cycle, also known as the Citric Acid Cycle or TCA Cycle, is a series of enzymatic reactions that take place in the mitochondrial matrix. It is a central part of cellular respiration, converting acetyl-CoA derived from carbohydrates, fats, and proteins into energy in the form of ATP, NADH, and FADH₂.
2. Key Steps in the Krebs Cycle
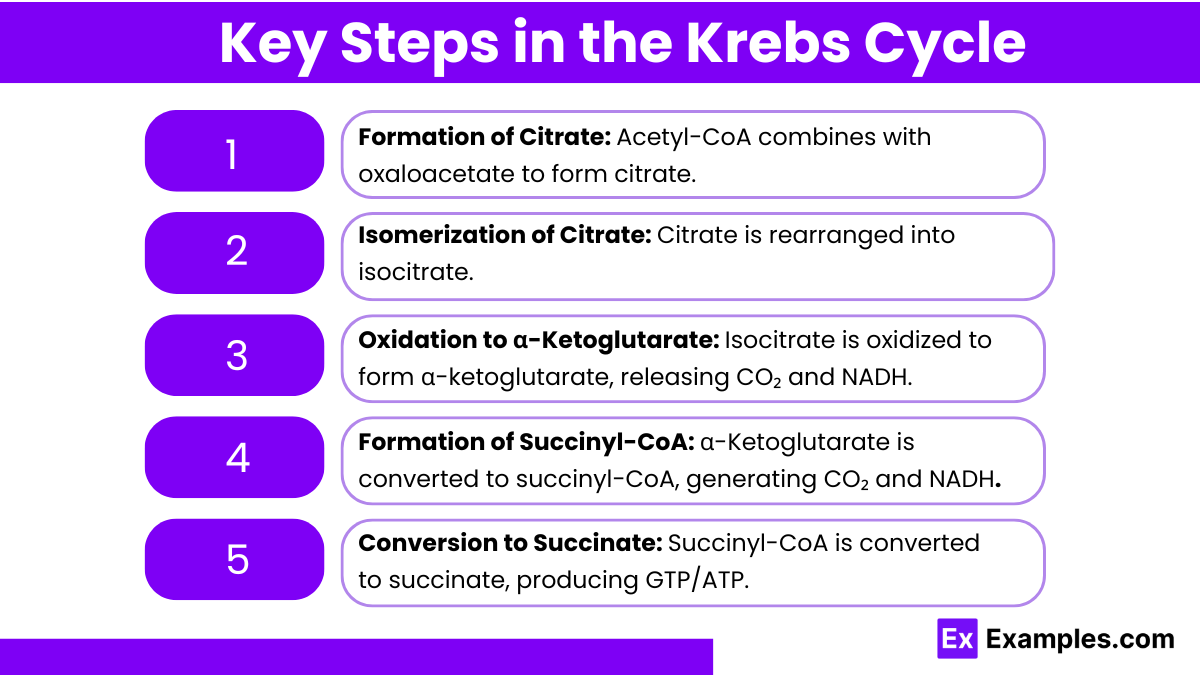
- Formation of Citrate:
- Acetyl-CoA (2-carbon) combines with oxaloacetate (4-carbon) to form citrate (6-carbon).
- Enzyme: Citrate synthase
- CoA is released in this step.
- Isomerization of Citrate:
- Citrate is converted into its isomer, isocitrate.
- Enzyme: Aconitase
- Oxidation to α-Ketoglutarate:
- Isocitrate is oxidized to α-ketoglutarate (5-carbon), releasing CO₂ and reducing NAD⁺ to NADH.
- Enzyme: Isocitrate dehydrogenase
- Formation of Succinyl-CoA:
- α-Ketoglutarate undergoes oxidative decarboxylation to form succinyl-CoA (4-carbon), releasing another CO₂ and reducing NAD⁺ to NADH.
- Enzyme: α-Ketoglutarate dehydrogenase
- Conversion to Succinate:
- Succinyl-CoA is converted to succinate, generating ATP (or GTP) through substrate-level phosphorylation.
- Enzyme: Succinyl-CoA synthetase
- Oxidation to Fumarate:
- Succinate is oxidized to fumarate, reducing FAD to FADH₂.
- Enzyme: Succinate dehydrogenase
- Conversion to Malate:
- Fumarate is hydrated to form malate.
- Enzyme: Fumarase
- Regeneration of Oxaloacetate:
- Malate is oxidized to oxaloacetate, reducing NAD⁺ to NADH, completing the cycle.
- Enzyme: Malate dehydrogenase
3. Energy Yield from Krebs Cycle
- For each acetyl-CoA molecule, the cycle produces:
- 3 NADH
- 1 FADH₂
- 1 ATP (or GTP)
- 2 CO₂
4. Oxidative Phosphorylation Overview
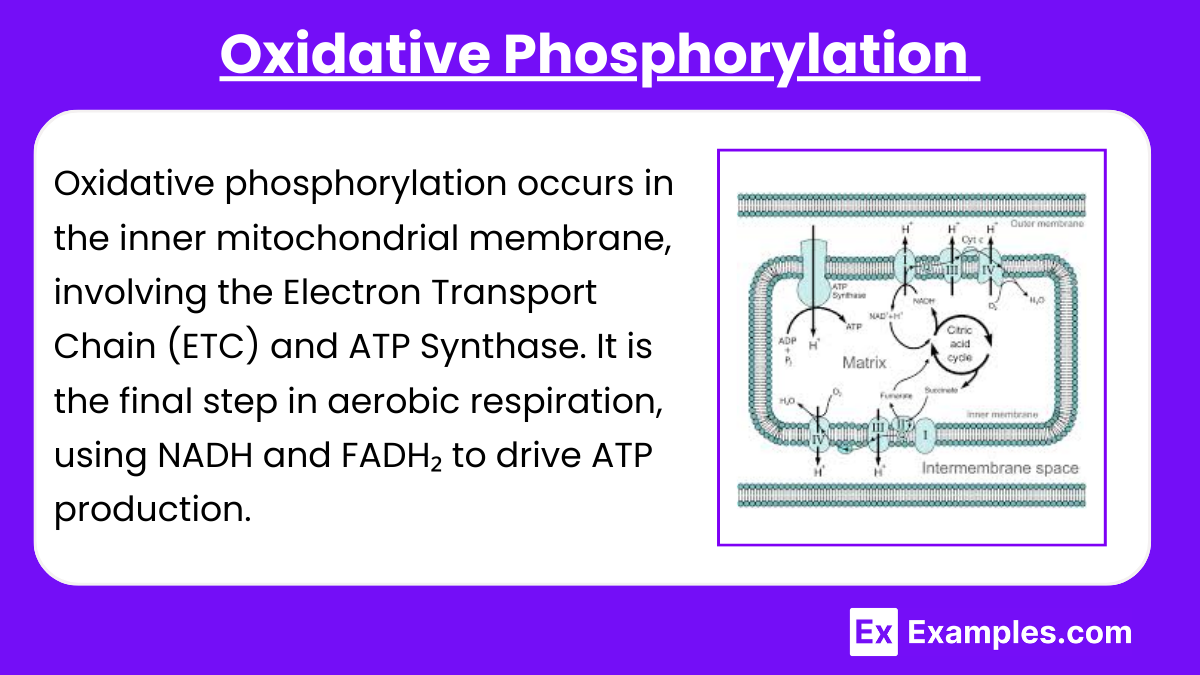
Oxidative phosphorylation occurs in the inner mitochondrial membrane, involving the Electron Transport Chain (ETC) and ATP Synthase. It is the final step in aerobic respiration, using NADH and FADH₂ to drive ATP production.
5. Key Steps in Oxidative Phosphorylation
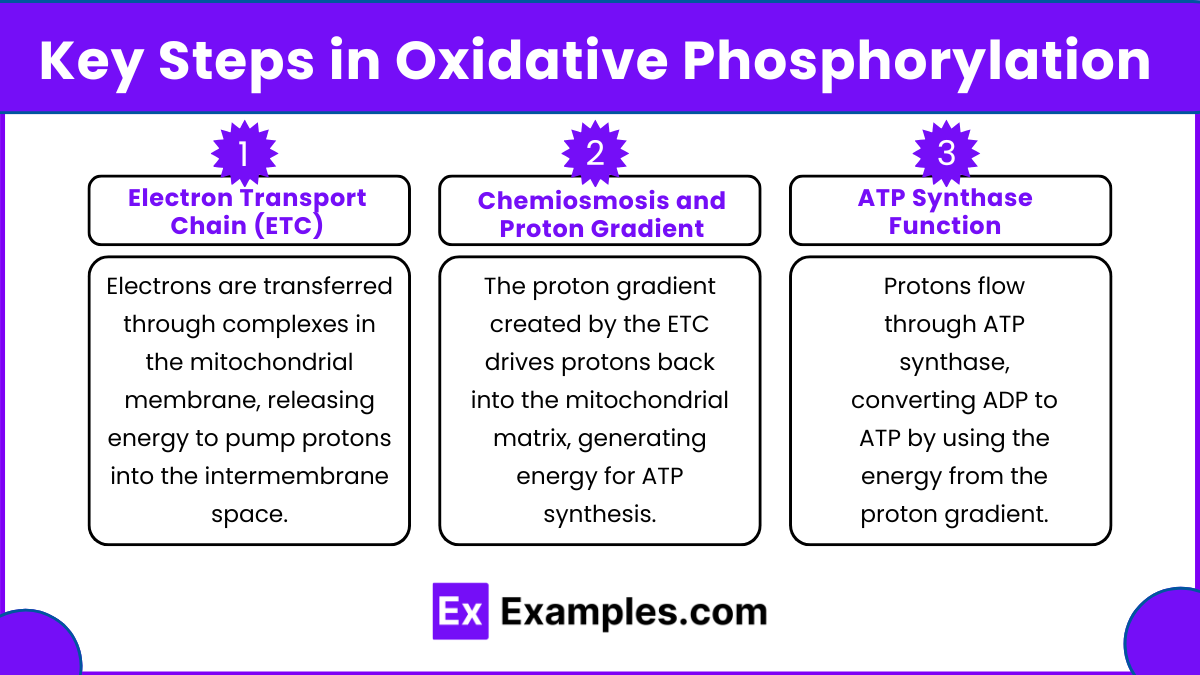
- Electron Transport Chain (ETC):
- The ETC consists of four protein complexes (Complexes I-IV), which facilitate the transfer of electrons from NADH and FADH₂ to oxygen, forming water.
- Complex I: Accepts electrons from NADH, pumping protons into the intermembrane space.
- Complex II: Accepts electrons from FADH₂; it does not pump protons.
- Complex III: Transfers electrons to cytochrome c and pumps protons.
- Complex IV: Transfers electrons to oxygen and pumps protons, forming water.
- Chemiosmosis and Proton Gradient:
- Protons are pumped from the mitochondrial matrix to the intermembrane space, creating a proton gradient.
- This gradient represents potential energy that drives ATP synthesis.
- ATP Synthase Function:
- ATP synthase uses the proton motive force to convert ADP and inorganic phosphate (Pi) into ATP.
- This process generates the bulk of ATP produced in aerobic respiration.
6. Energy Yield from Oxidative Phosphorylation
- NADH generates approximately 2.5 ATP, while FADH₂ generates about 1.5 ATP.
- In total, oxidative phosphorylation produces around 26-28 ATP molecules per glucose molecule.
Regulation of Krebs Cycle and Oxidative Phosphorylation
- Krebs Cycle:
- Regulated by the availability of substrates (e.g., NAD⁺, ADP) and feedback inhibition (e.g., NADH).
- Key regulatory enzymes include citrate synthase, isocitrate dehydrogenase, and α-ketoglutarate dehydrogenase.
- Oxidative Phosphorylation:
- Regulated primarily by the availability of ADP and oxygen.
- The proton gradient is a key factor in controlling ATP synthesis.
Examples
Example 1. Energy Production in Muscle Cells during Exercise
During intense physical activity, muscle cells require a high amount of ATP to sustain contractions. The Krebs cycle, occurring in the mitochondria, plays a crucial role by breaking down acetyl-CoA derived from carbohydrates, fats, and proteins, generating NADH and FADH₂. These electron carriers feed into the oxidative phosphorylation pathway, driving ATP synthesis. This efficient energy production enables muscles to perform sustained activities, like running or weightlifting.
Example 2. Glucose Metabolism in the Liver:
In the liver, glucose is metabolized through glycolysis to produce pyruvate, which is converted to acetyl-CoA and enters the Krebs cycle. Here, the breakdown of acetyl-CoA generates electron carriers that subsequently enter oxidative phosphorylation to produce ATP. This process supports various liver functions, including detoxification, urea production, and the synthesis of vital proteins, ensuring the liver meets its energy demands even during fasting or low glucose intake.
Example 3. Fatty Acid Oxidation in Adipose Tissue:
Adipose tissue, or fat cells, stores triglycerides, which are broken down into free fatty acids and glycerol during periods of low energy intake. These fatty acids are transported to the mitochondria, where they undergo beta-oxidation to form acetyl-CoA. This acetyl-CoA enters the Krebs cycle, leading to the production of NADH and FADH₂, which drive oxidative phosphorylation. This process allows fat cells to produce ATP, supporting energy needs during prolonged fasting or caloric deficits.
Example 4. Aerobic Respiration in Brain Cells:
Neurons in the brain are highly energy-dependent and primarily rely on glucose metabolism to sustain their activities. Glucose is broken down through glycolysis and subsequently enters the Krebs cycle as acetyl-CoA. The cycle generates NADH and FADH₂, which fuel oxidative phosphorylation, producing the ATP necessary for neurotransmitter synthesis, signal transmission, and maintaining ion gradients across neuronal membranes, supporting overall cognitive function.
Example 5. Oxidative Stress Management in Heart Cells:
The heart is an organ with a constant demand for ATP to maintain its rhythmic contractions. Cardiac muscle cells depend heavily on the Krebs cycle and oxidative phosphorylation for ATP production, using substrates like fatty acids, glucose, and lactate. Proper functioning of this pathway is also vital in managing oxidative stress, as the controlled production of reactive oxygen species (ROS) occurs alongside ATP synthesis. The balance between ATP production and ROS management is crucial for the heart’s endurance and efficiency, especially during physical exertion or stress.
Practice Questions
Question 1
Which of the following is a direct product of the Krebs cycle that enters the electron transport chain?
A) Acetyl-CoA
B) NADH
C) Pyruvate
D) Glucose
Correct Answer: B) NADH
Explanation:
The Krebs cycle (also known as the citric acid cycle) is a crucial metabolic pathway that occurs in the mitochondrial matrix. During this cycle, energy-rich molecules like NADH and FADH₂ are generated. These molecules are electron carriers that transfer electrons to the electron transport chain (ETC) for oxidative phosphorylation. While Acetyl-CoA is the initial substrate that enters the Krebs cycle, it does not enter the ETC directly. Similarly, pyruvate is converted into Acetyl-CoA before entering the Krebs cycle, and glucose is broken down in glycolysis before entering the cycle.
Question 2
During oxidative phosphorylation, the final electron acceptor in the electron transport chain is:
A) NAD+
B) ADP
C) O₂ (oxygen)
D) CO₂ (carbon dioxide)
Correct Answer: C) O₂ (oxygen)
Explanation:
In oxidative phosphorylation, electrons move through a series of protein complexes in the inner mitochondrial membrane (known as the electron transport chain). At the end of this chain, oxygen (O₂) serves as the final electron acceptor. It combines with electrons and protons (H⁺) to form water (H₂O). This step is essential for maintaining the flow of electrons through the chain, as the accumulation of electrons would halt the process if not accepted by oxygen. The other options—NAD+, ADP, and CO₂—are not involved as final electron acceptors.
Question 3
How many ATP molecules are produced from one molecule of glucose during the Krebs cycle and oxidative phosphorylation combined?
A) 2 ATP
B) 4 ATP
C) 18 ATP
D) 30-32 ATP
Correct Answer: D) 30-32 ATP
Explanation:
One glucose molecule undergoes glycolysis, the Krebs cycle, and oxidative phosphorylation, leading to the production of 30-32 ATP molecules in total. Here’s how this breakdown occurs:
- Krebs cycle: Directly produces 2 ATP through substrate-level phosphorylation and generates 6 NADH and 2 FADH₂, which are used in oxidative phosphorylation.
- Oxidative phosphorylation: Each NADH can yield about 2.5 ATP, while each FADH₂ yields about 1.5 ATP. Considering NADH and FADH₂ from glycolysis, pyruvate oxidation, and the Krebs cycle, the combined yield is 26-28 ATP from oxidative phosphorylation.
- Overall, adding ATP from the Krebs cycle and oxidative phosphorylation leads to the 30-32 ATP range.