“Light and electromagnetic radiation encompass a spectrum of waves, including visible light, UV, infrared, and radio waves. Understanding wave-particle duality, energy quantization, and interactions with matter is essential for analyzing phenomena like reflection, refraction, absorption, and emission relevant to MCAT physics and chemistry.”
Learning Objectives
In studying “Light and Electromagnetic Radiation” for the MCAT, you should learn to understand the properties of electromagnetic waves, including their wavelength, frequency, speed, and energy. Differentiate between the electromagnetic spectrum regions, such as visible light, ultraviolet, infrared, and X-rays, and their applications in biological and medical contexts. Explore the dual nature of light, behaving both as a particle (photon) and a wave. Analyze how light interacts with matter through reflection, refraction, diffraction, and interference. Apply the concepts of Snell’s law and the index of refraction in problem-solving. Understand the principles of polarization and the Doppler effect in relation to electromagnetic waves. Additionally, learn to evaluate how electromagnetic radiation impacts biological systems, such as in vision, photosynthesis, and medical imaging technologies like X-rays and MRIs.
Understanding the Properties of Electromagnetic Waves
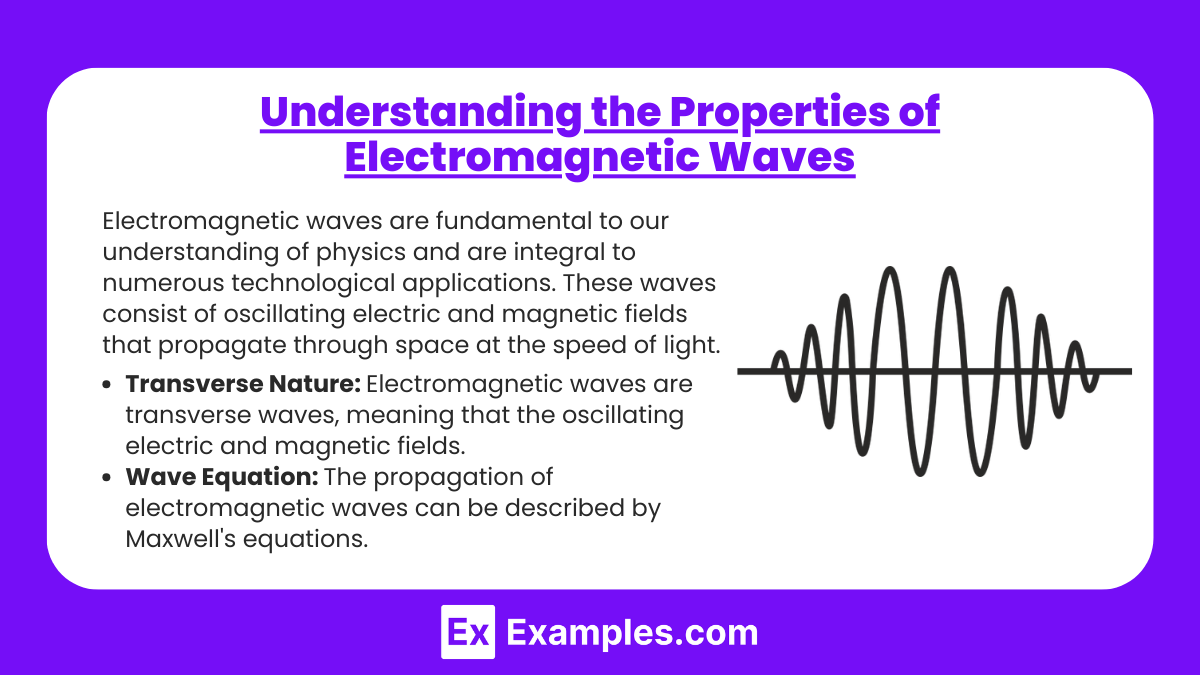
Electromagnetic waves are fundamental to our understanding of physics and are integral to numerous technological applications. These waves consist of oscillating electric and magnetic fields that propagate through space at the speed of light, which is approximately 3.00×108 meters per second in a vacuum. They require no medium to travel, differentiating them from mechanical waves such as sound.
Key Properties of Electromagnetic Waves
- Transverse Nature: Electromagnetic waves are transverse waves, meaning that the oscillating electric and magnetic fields are perpendicular to each other and to the direction of wave propagation. If the wave travels in the z-direction, the electric field might oscillate in the x-direction, and the magnetic field in the y-direction, for example.
- Wave Equation: The propagation of electromagnetic waves can be described by Maxwell’s equations, which predict that changes in electric and magnetic fields propagate outward at the speed of light.
- Speed of Light:
- The speed of light in vacuum (c) is one of the fundamental constants and is related to the permittivity and permeability of free space.
- This speed represents the maximum speed at which all energy, matter, and information in the universe can travel.
- Energy Transport:
- Electromagnetic waves carry energy as they propagate through space. The energy carried by an electromagnetic wave is proportional to the square of the amplitude of the fields.
- Momentum and Pressure:
- Electromagnetic waves also carry momentum, which can exert pressure when the waves interact with matter (radiation pressure). This is exploited in technologies like solar sails for spacecraft propulsion.
Spectrum of Electromagnetic Waves
Electromagnetic waves cover a broad spectrum of wavelengths and frequencies. From longest wavelength to shortest, this spectrum includes:
- Radio Waves: Used for broadcasting and communications.
- Microwaves: Used in microwave ovens, radar technology, and certain types of communication.
- Infrared Radiation: Associated with heat; used in heaters, night-vision equipment, and remote controls.
- Visible Light: The only part of the spectrum visible to the human eye, used in a plethora of applications from lighting to photography.
- Ultraviolet Light: Has applications in sterilization, fluorescent lamps, and detecting counterfeit money.
- X-rays: Used in medical imaging and security scanners.
- Gamma Rays: Emitted by nuclear reactions and certain astronomical events, used in cancer treatment and astrophysics.
Differentiating Between Regions of the Electromagnetic Spectrum
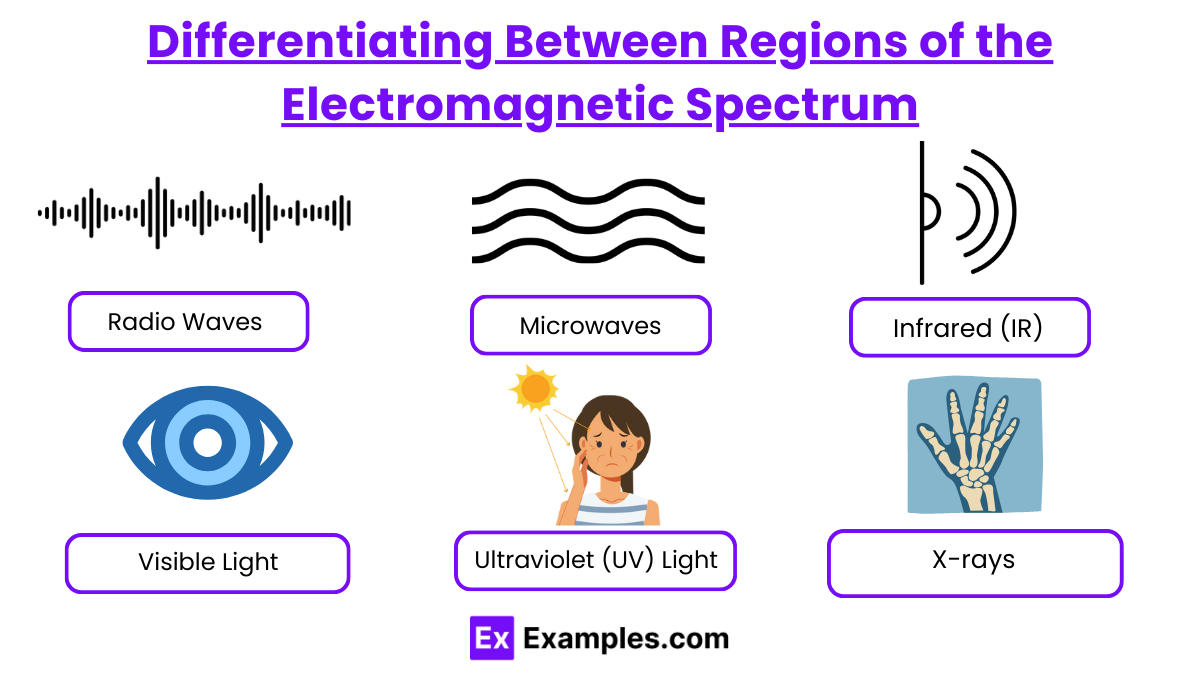
The electromagnetic (EM) spectrum encompasses a range of electromagnetic waves that vary in wavelength and frequency. This spectrum is typically divided into regions based on the wavelength, frequency, and energy of the waves. Each region has unique characteristics and practical applications that are integral to modern technology, medicine, and science.
Overview of the Electromagnetic Spectrum Regions
Here is a brief overview of each region, from the longest to the shortest wavelengths:
- Radio Waves
- Wavelength: Longer than 1 millimeter (mm)
- Frequency: Below 300 gigahertz (GHz)
- Applications: Communication (radio, television, mobile phones), astronomy, and navigation.
- Microwaves
- Wavelength: 1 mm to 1 meter (m)
- Frequency: 300 MHz to 300 GHz
- Applications: Microwave ovens, radar, satellite communications, and Wi-Fi.
- Infrared (IR)
- Wavelength: 700 nanometers (nm) to 1 mm
- Frequency: 300 GHz to 430 terahertz (THz)
- Applications: Heat sensors, night vision equipment, remote controls, and thermal imaging.
- Visible Light
- Wavelength: 400 nm to 700 nm
- Frequency: 430 THz to 790 THz
- Applications: All applications involving sight, photography, illumination.
- Ultraviolet (UV) Light
- Wavelength: 10 nm to 400 nm
- Frequency: 790 THz to 30 petahertz (PHz)
- Applications: Sterilization, fluorescent lamps, detecting forged bank notes, and tanning.
- X-rays
- Wavelength: 0.01 nm to 10 nm
- Frequency: 30 PHz to 30 exahertz (EHz)
- Applications: Medical imaging, cancer treatment, airport security, and crystallography.
- Gamma Rays
- Wavelength: Less than 0.01 nm
- Frequency: Greater than 30 EHz
- Applications: Astrophysics, radiation therapy, and nuclear energy.
Differentiating Factors
- Wavelength and Frequency: Each region of the EM spectrum can be differentiated based on its wavelength and frequency. Generally, as the frequency increases, the wavelength decreases. This inverse relationship dictates the energy properties of the waves, with higher frequencies having higher energies.
- Energy: The energy of electromagnetic waves increases as you move from radio waves to gamma rays. This is because energy is directly proportional to frequency.
- Interaction with Matter: How these waves interact with matter also varies. For example:
- Radio waves can penetrate non-conductive materials like plastic and wood.
- Microwaves are absorbed by water and fats, which is why they are useful for cooking.
- Infrared radiation is absorbed and emitted by objects as heat.
- Visible light is the only part of the spectrum that can be detected by the human eye.
- Ultraviolet light can cause chemical reactions, like skin tanning.
- X-rays can penetrate most substances but are absorbed by bones and metals, which makes them useful for imaging.
- Gamma rays, being the most energetic, can penetrate almost all materials and are used to kill cancer cells and sterilize medical equipment.
Exploring the Dual Nature of Light: Wave and Particle
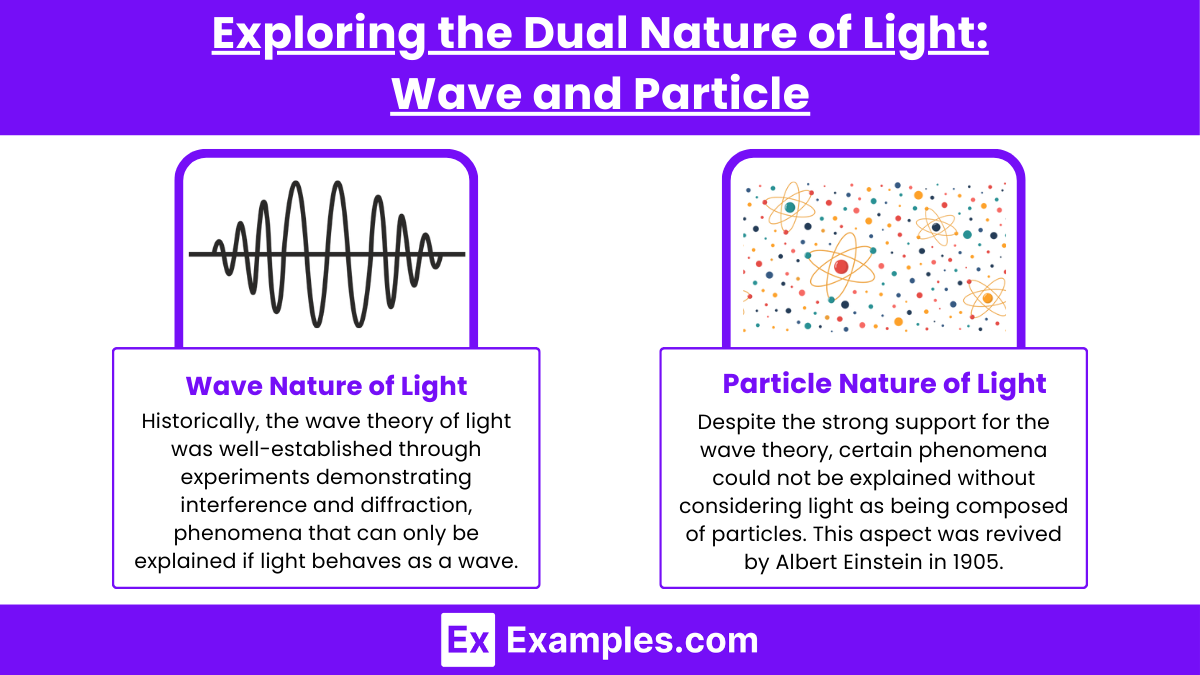
The dual nature of light is a fundamental concept in modern physics, revealing that light exhibits both wave-like and particle-like behaviors. This duality is one of the core principles of quantum mechanics and has profound implications for our understanding of light and energy.
Wave Nature of Light
Historically, the wave theory of light was well-established through experiments demonstrating interference and diffraction, phenomena that can only be explained if light behaves as a wave.
Key Experiments and Concepts:
- Thomas Young’s Double-Slit Experiment (1801): Demonstrated interference patterns when light passed through two closely spaced slits, creating bright and dark bands on a screen. This effect, which arises from constructive and destructive interference of waves, strongly supported the wave theory of light.
- Maxwell’s Equations (1865): James Clerk Maxwell formulated equations that described light as electromagnetic waves, comprising oscillating electric and magnetic fields traveling through space. These waves predicted that light travels at a constant speed, which matches the speed measured for electromagnetic radiation.
Particle Nature of Light
Despite the strong support for the wave theory, certain phenomena could not be explained without considering light as being composed of particles. This aspect was revived by Albert Einstein in 1905.
Key Experiments and Concepts:
- Photoelectric Effect: Einstein explained the photoelectric effect, where light incident on a material can effect electrons from its surface. The wave theory could not explain why the energy of ejected electrons was dependent on the light’s frequency, not its intensity. Einstein proposed that light consists of quantized packets of energy, called photons, which have energy proportional to their frequency (E = hν), where h is Planck’s constant and ν\nuν is the frequency of light. This explanation earned him the Nobel Prize in Physics in 1921.
- Compton Scattering (1923): Arthur Compton showed that X-rays scatter off electrons as if they were particles, with the X-rays transferring part of their energy and momentum to the electrons. This scattering could be perfectly predicted by treating X-rays as particles, further confirming the particle nature of light.
Wave-Particle Duality
The concept of wave-particle duality emerged to reconcile the wave and particle theories of light. This principle asserts that light can exhibit both wave-like and particle-like properties, depending on the experimental setup.
- Quantum Mechanics: Modern quantum mechanics describes light and matter as having characteristics of both particles and waves. Photons are treated as quantum objects that exhibit wave-like interference and diffraction, as well as particle-like behavior in interactions such as the photoelectric effect.
- Heisenberg Uncertainty Principle: This principle in quantum mechanics highlights that certain pairs of physical properties, like position and momentum, cannot be simultaneously measured with arbitrarily high precision. This principle underlines the fundamental limits imposed by the dual nature of light.
Understanding Polarization and the Doppler Effect
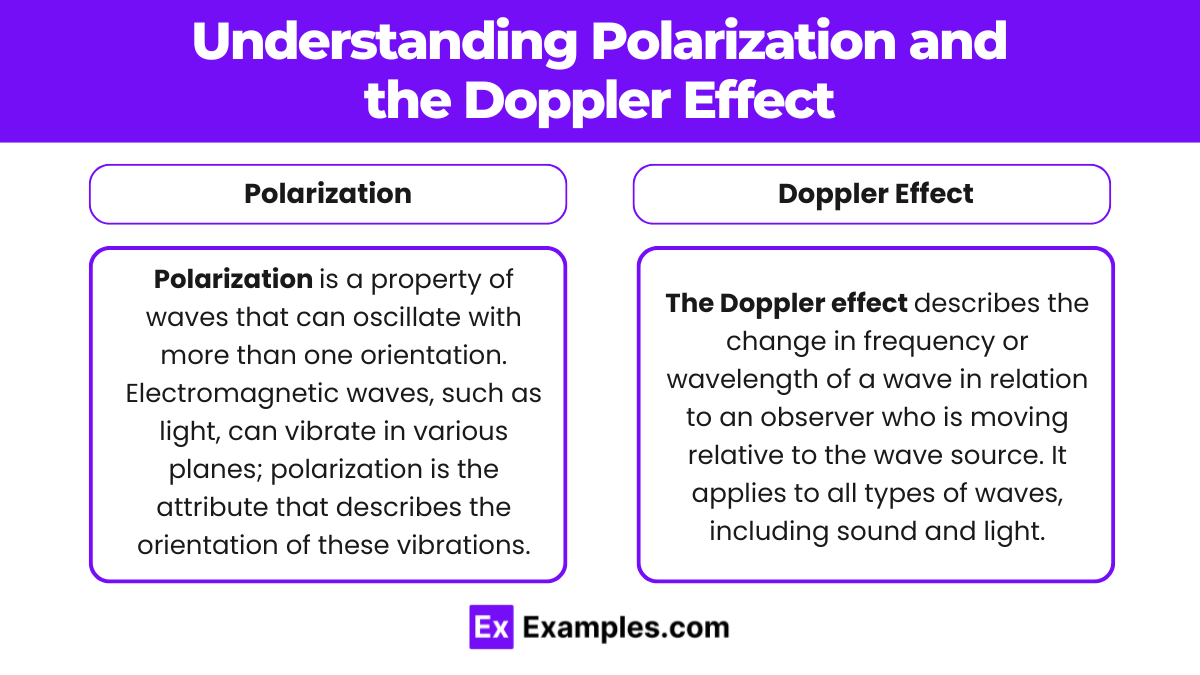
Understanding polarization and the Doppler effect involves delving into two different, yet fundamental aspects of wave behavior. Polarization pertains to the orientation of wave oscillations, relevant primarily to transverse waves like light and certain mechanical waves, while the Doppler effect involves changes in frequency and wavelength due to the relative motion between the source of waves and an observer.
Polarization
Polarization is a property of waves that can oscillate with more than one orientation. Electromagnetic waves, such as light, can vibrate in various planes; polarization is the attribute that describes the orientation of these vibrations.
Types of Polarization:
- Linear Polarization: The electric field of light vibrates in one plane along the direction of propagation. This is common in many light sources.
- Circular Polarization: The electric field of the light wave rotates in a circle around the direction of propagation, which can be right-handed or left-handed.
- Elliptical Polarization: A general form of polarization where the electric field describes an ellipse in the plane perpendicular to the direction of propagation. This form encompasses both linear and circular as special cases.
Applications of Polarization:
- Optics: Polarized sunglasses reduce glare by blocking horizontally polarized light.
- Photography: Polarizing filters enhance pictures by reducing reflections and enhancing the sky’s contrast against clouds.
- Communication: Polarization is used in satellite and radio communications to reduce interference between signals.
Doppler Effect
The Doppler effect describes the change in frequency or wavelength of a wave in relation to an observer who is moving relative to the wave source. It applies to all types of waves, including sound and light.
Key Concepts:
- Approaching Source: When the source of the wave moves towards the observer, the wavelengths become shorter, and the frequency increases (blue shift in light).
- Receding Source: When the source moves away from the observer, the wavelengths become longer, and the frequency decreases (red shift in light).
Applications of the Doppler Effect:
- Astronomy: The Doppler effect is used to determine whether stars and galaxies are moving towards or away from us, indicating their speed and direction. This is vital in the study of the expanding universe.
- Radar: Used by police to detect speeding vehicles by measuring the change in frequency of reflected radio waves.
- Medical Imaging: Doppler ultrasound measures changes in frequency to visualize blood flow or other moving body parts, helping diagnose various conditions.
Examples
Example 1: Visible Light Spectrum
Capacitors are commonly used in power supply circuits to smooth out voltage fluctuations. When alternating current (AC) is converted to direct current (DC), capacitors store excess charge and release it when the voltage dips, ensuring a more stable output. This smoothing action helps prevent voltage spikes and provides a consistent power supply for electronic devices.
Example 2: Ultraviolet (UV) Radiation
In timing applications, capacitors work in conjunction with resistors to create delays. For example, in a simple RC (resistor-capacitor) timing circuit, a capacitor charges and discharges at a rate determined by the resistance and capacitance values. This principle is used in various applications, such as generating time delays in blinking LED circuits or timing events in digital devices.
Example 3: Infrared (IR) Radiation
Capacitors are essential components in audio equipment, where they serve as filters. In crossover networks for speakers, capacitors can block certain frequencies while allowing others to pass, ensuring that high-frequency signals reach tweeters and low-frequency signals reach woofers. This filtering enhances sound quality and protects speakers from damage due to inappropriate frequency ranges.
Example 4: X-rays
Capacitors are used in camera flash systems to store energy and release it quickly for a brief, intense burst of light. When the flash is triggered, the stored energy in the capacitor discharges rapidly through the flash bulb, providing the necessary brightness for capturing well-lit images in low-light conditions. This rapid discharge highlights the capacitor’s ability to store and release energy efficiently.
Example 5: Radio Waves
In electric motors, especially single-phase motors, capacitors help start the motor by providing an initial boost of energy. The motor start capacitor stores energy and releases it to create a phase shift in the current, allowing the motor to start smoothly and efficiently. Once the motor reaches a certain speed, the capacitor is typically disconnected from the circuit, demonstrating its role in enhancing motor performance.
Practice Questions
Question 1
Which of the following correctly describes the nature of light?
A) Light travels in straight lines and behaves only as a particle.
B) Light is a form of electromagnetic radiation that exhibits both wave-like and particle-like properties.
C) Light cannot travel through a vacuum and requires a medium.
D) Light has a fixed speed regardless of the observer’s frame of reference.
Correct Answer: B) Light is a form of electromagnetic radiation that exhibits both wave-like and particle-like properties.
Explanation: Light is best understood as electromagnetic radiation that exhibits dual characteristics: it can behave as a wave (demonstrating phenomena such as interference and diffraction) and as a particle (exhibiting properties of photons). Option A is incorrect because light also shows wave behavior. Option C is false since light can travel through a vacuum, and option D is incorrect as the speed of light remains constant at approximately 299,792 kilometers per second in a vacuum, regardless of the observer’s motion.
Question 2
What phenomenon occurs when light passes through a prism?
A) Reflection
B) Diffraction
C) Refraction
D) Absorption
Correct Answer: C) Refraction.
Explanation: When light passes through a prism, it bends due to refraction, which occurs as light travels from one medium (air) into another medium (glass) with a different density. This bending causes the different wavelengths (colors) of light to separate, resulting in a spectrum. Option A refers to light bouncing off a surface, option B pertains to the spreading of waves when they pass through a narrow opening, and option D involves light being absorbed by a material, none of which describe the separation of colors that occurs in a prism.
Question 3
Which of the following statements about electromagnetic radiation is true?
A) All electromagnetic waves travel at different speeds in a vacuum.
B) The wavelength of electromagnetic radiation is inversely proportional to its frequency.
C) Electromagnetic radiation can only be seen by the human eye.
D) X-rays have a longer wavelength than radio waves.
Correct Answer: B) The wavelength of electromagnetic radiation is inversely proportional to its frequency.
Explanation: In electromagnetic radiation, the relationship between wavelength and frequency is such that as the frequency increases, the wavelength decreases, and vice versa. This inverse relationship is fundamental to understanding how different types of electromagnetic radiation, such as radio waves, visible light, and X-rays, are categorized. Option A is incorrect because all electromagnetic waves travel at the same speed in a vacuum. Option C is false because electromagnetic radiation includes a wide spectrum beyond visible light, such as ultraviolet and infrared radiation. Option D is also incorrect since X-rays have a shorter wavelength than radio waves.