Magnetism, a key topic in the MCAT, explores the forces exerted by magnetic fields on moving charges and currents. It covers magnetic fields, electromagnetism, the motion of charged particles, right-hand rules, and applications such as MRI technology and electromagnetic induction.
Learning Objectives
In studying “Magnetism” for the MCAT, you should learn to understand the properties and sources of magnetic fields, including how moving charges and currents generate magnetic fields. Explore the relationship between electric currents and magnetic forces using the right-hand rule. Analyze how charged particles behave in magnetic fields, including circular and helical motion. Understand the concept of magnetic flux and Faraday’s law of electromagnetic induction, which explains how changing magnetic fields induce electric currents. Evaluate the workings of key devices like solenoids and electromagnets in generating magnetic fields. Additionally, understand the biological relevance of magnetism, such as how magnetic resonance imaging (MRI) applies these principles in medical diagnostics.
Properties and Sources of Magnetic Fields
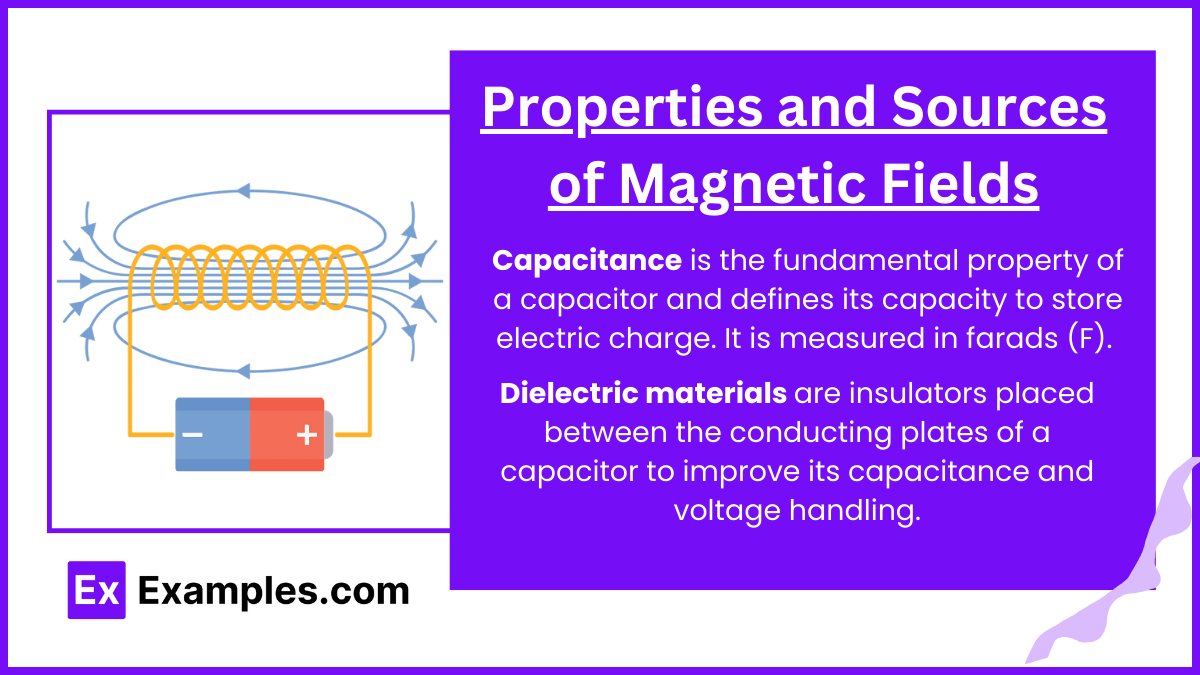
Capacitance
Capacitance is the fundamental property of a capacitor and defines its capacity to store electric charge. It is measured in farads (F), a unit that quantifies how much electric charge is stored per volt of electrical potential applied across the capacitor.
Determining Factors:
- Area of the Plates (A): The capacitance is directly proportional to the area of the conducting plates. Larger plate areas provide more space for storing charge, thus increasing capacitance.
- Distance Between the Plates (d): Capacitance is inversely proportional to the distance between the plates. Closer plates result in a stronger electric field for a given charge, increasing capacitance.
- Dielectric Constant (κ): The material between the plates, known as the dielectric, also affects capacitance. The dielectric constant of the material is a measure of its ability to increase the capacitance compared to empty space (vacuum).
The capacitance of a parallel-plate capacitor can be calculated with the formula: C = κϵ0A/d
where:
- ϵ0 is the permittivity of free space (approximately 8.85×10−12,
- κ is the relative permittivity or dielectric constant of the material between the plates,
- A is the area of one of the plates,
- d is the separation between the two plates.
Dielectric Materials
Dielectric materials are insulators placed between the conducting plates of a capacitor to improve its capacitance and voltage handling. The choice of dielectric material impacts not only the capacitance but also other crucial factors such as the capacitor’s size, temperature stability, and frequency response.
Key Properties of Dielectric Materials:
- Dielectric Constant (Relative Permittivity): A higher dielectric constant means the material can store more charge at a given voltage, effectively increasing the capacitance.
- Dielectric Strength: This is the maximum electric field a dielectric material can withstand without breaking down. Higher dielectric strength allows a capacitor to operate at higher voltages.
- Loss Tangent (Dissipation Factor): This property measures how much energy from the electric field is lost as heat within the dielectric. Lower values indicate more efficient capacitors with minimal energy losses.
Common Dielectric Materials:
- Ceramic: Used in ceramic capacitors, offering high frequency characteristics and good temperature stability. They are commonly used in high-frequency applications like RF circuitry.
- Polyester and Polypropylene: These plastics are used in film capacitors and are valued for their stability and low cost. They are commonly used in power systems and audio electronics.
- Tantalum: Tantalum capacitors have high capacitance values and are very stable, making them ideal for filtering applications in power supply circuits in compact electronic devices.
- Electrolytic: Aluminum and tantalum electrolytic capacitors have a very high capacitance-to-volume ratio, suited for applications requiring large capacitance values, such as in power smoothing circuits.
Electric Currents and Magnetic Forces: Using the Right-Hand Rule
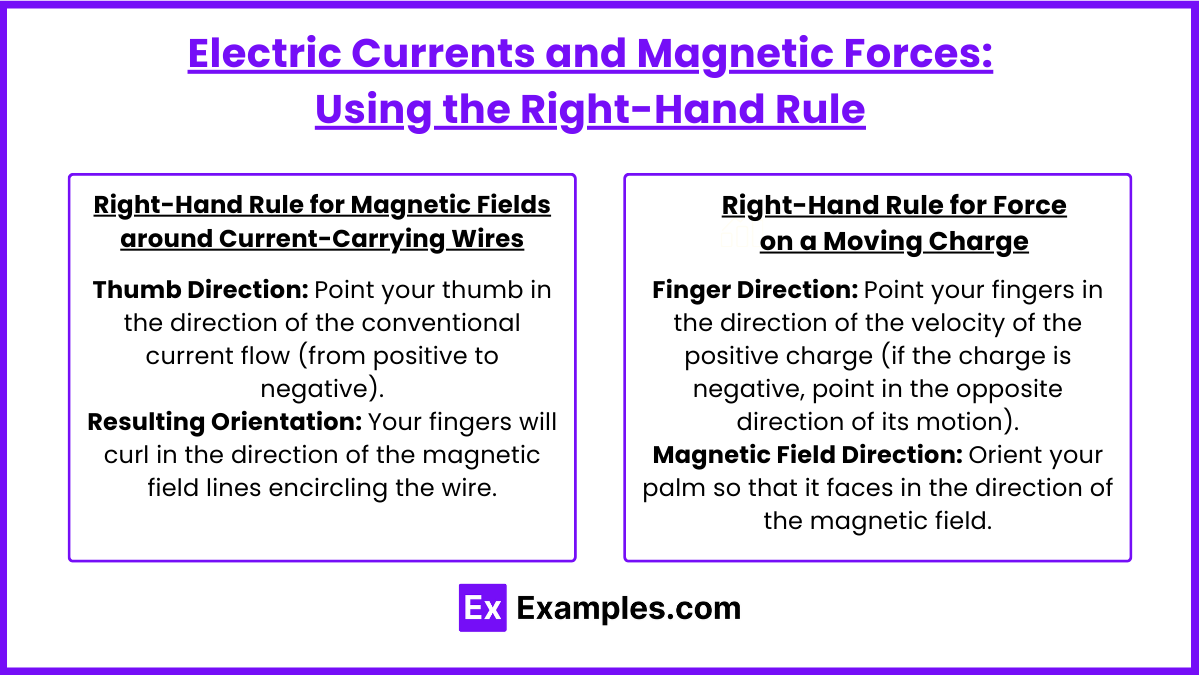
Electric currents produce magnetic fields, and the relationship between them is foundational to electromagnetism. A useful way to determine the direction of the magnetic field generated by an electric current, or the force experienced by a charged particle moving in a magnetic field, is through the “Right-Hand Rule.” This rule is a simple mnemonic that helps visualize the directions involved in electromagnetic interactions.
Right-Hand Rule for Magnetic Fields around Current-Carrying Wires
When an electric current flows through a wire, it creates a magnetic field that circles around the wire. The direction of this magnetic field can be determined using the right-hand rule:
- Thumb Direction: Point your thumb in the direction of the conventional current flow (from positive to negative).
- Fingers Curl: Curl your fingers around the wire.
- Resulting Orientation: Your fingers will curl in the direction of the magnetic field lines encircling the wire.
This rule applies to straight wires, solenoids, and loops. For loops or coils (solenoids), the curled fingers represent the loops of the coil, and the thumb points in the direction of the magnetic field inside the coil.
Right-Hand Rule for Force on a Moving Charge
When a charged particle moves through a magnetic field, it experiences a force called the Lorentz force. The direction of this force can also be determined using a variation of the right-hand rule:
- Finger Direction: Point your fingers in the direction of the velocity of the positive charge (if the charge is negative, point in the opposite direction of its motion).
- Magnetic Field Direction: Orient your palm so that it faces in the direction of the magnetic field.
- Thumb Direction: Raise your thumb. It will now point in the direction of the force exerted on a positive charge. For a negative charge, the force direction will be opposite to the thumb.
This rule helps in understanding the behavior of particles in devices like cyclotrons and mass spectrometers, as well as in everyday technology such as electric motors.
Devices Generating Magnetic Fields: Solenoids and Electromagnets
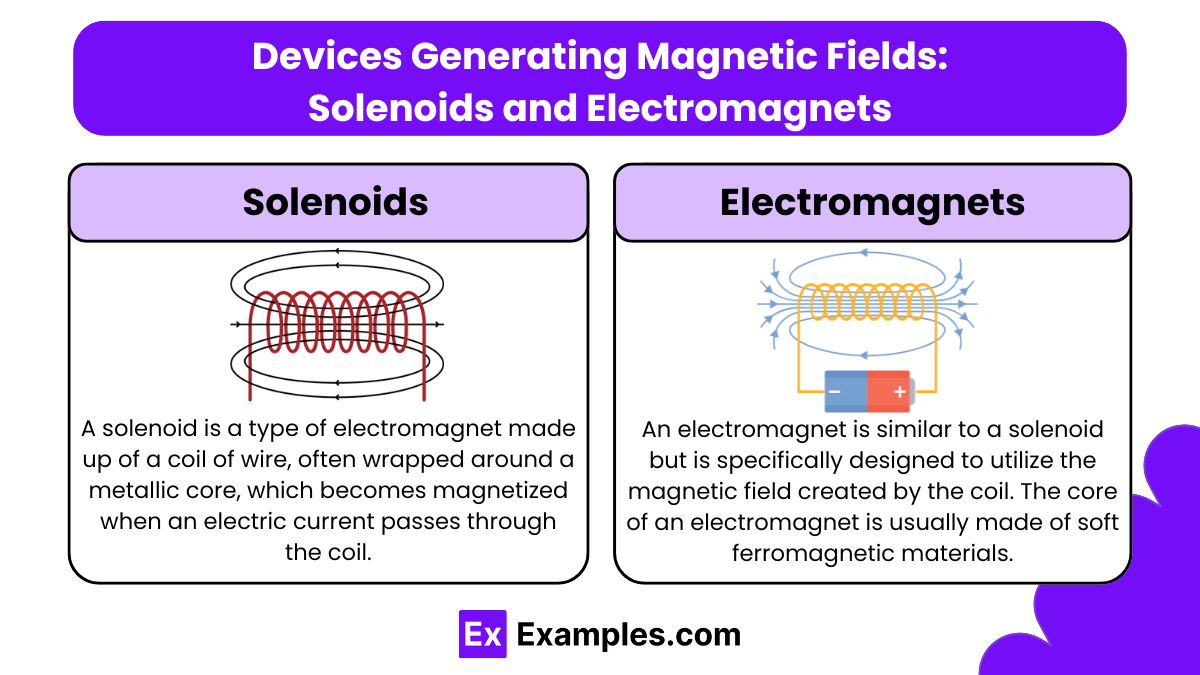
Devices that generate magnetic fields, such as solenoids and electromagnets, are pivotal in various applications, ranging from industrial machinery to everyday electronic devices. Both solenoids and electromagnets use electric currents to produce controlled magnetic fields, leveraging the principles of electromagnetism to achieve specific functionalities.
Solenoids
A solenoid is a type of electromagnet made up of a coil of wire, often wrapped around a metallic core, which becomes magnetized when an electric current passes through the coil.
Construction and Operation:
- Coil: The solenoid consists of a long wire wound into a helical shape. The coil turns can be closely packed or have some spacing, depending on the design requirements.
- Core: While solenoids can operate without a core, inserting a ferromagnetic core (like iron) inside the coil greatly enhances the magnetic field’s strength and focus.
Magnetic Field Generation:
- When current flows through the solenoid, it generates a magnetic field with lines of force running parallel inside the coil, creating a strong uniform field. The field resembles that of a bar magnet, with a distinct north and south pole at each end of the coil.
Applications:
- Valves: Solenoids actuate valves in various systems, controlling fluid flow in applications like washing machines, hydraulic systems, and industrial processing.
- Automotive Starters: Solenoids are used in car starters, where the solenoid acts as both a relay and a device to engage the starter gear.
- Electromechanical Relays and Switches: Used to control large power loads with minimal input power, solenoids can make or break an electrical connection mechanically.
Electromagnets
An electromagnet is similar to a solenoid but is specifically designed to utilize the magnetic field created by the coil. The core of an electromagnet is usually made of soft ferromagnetic materials, which enhances the magnetic field but does not retain magnetism after the current is switched off.
Construction and Operation:
- Coil: Just like in solenoids, the electromagnet consists of a coil of wire. The shape and size of the coil depend on its intended use.
- Core: Typically made from iron or its alloys, which is optimized to enhance the magnetic field strength temporarily when an electric current flows through the coil.
Applications:
- Cranes for Moving Scrap Metal: Large electromagnets are used in junkyards and recycling centers to move large pieces of metal or scrap.
- MRI Machines: Electromagnets are crucial in the healthcare industry, particularly in MRI machines, where they are used to generate a strong magnetic field necessary for imaging internal body structures.
- Electric Bells and Buzzers: These devices use an electromagnet to rapidly move a mechanical striker.
Biological Relevance of Magnetism: Magnetic Resonance Imaging (MRI)
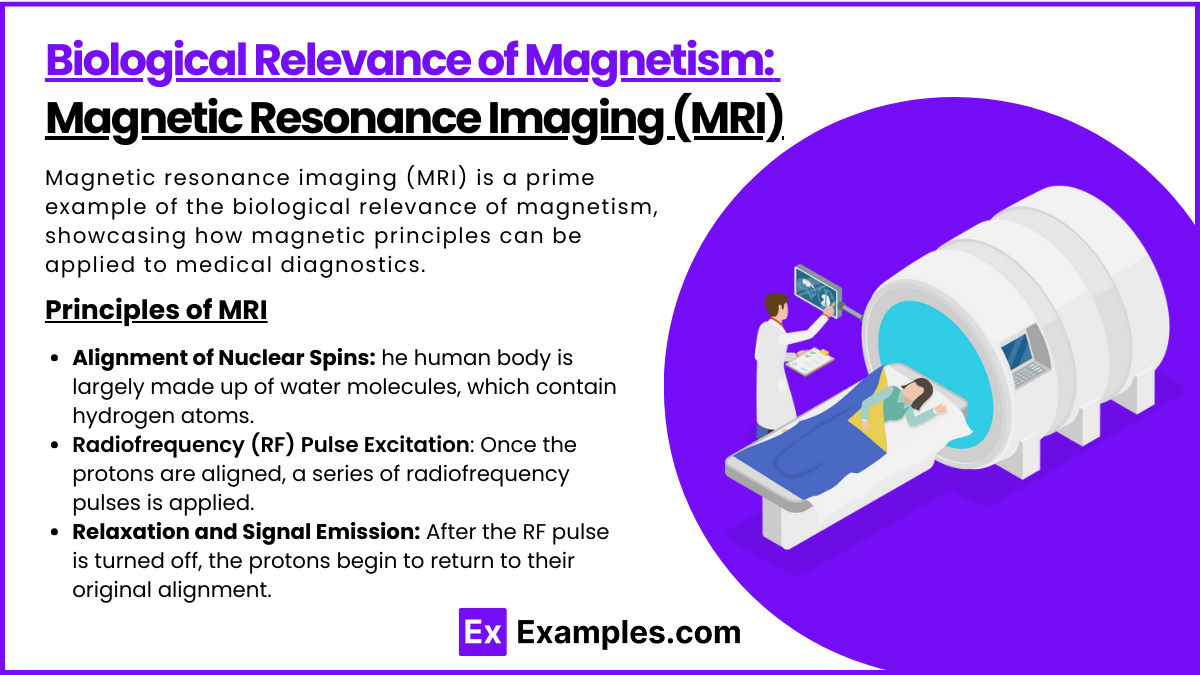
Magnetic resonance imaging (MRI) is a prime example of the biological relevance of magnetism, showcasing how magnetic principles can be applied to medical diagnostics. MRI uses strong magnetic fields and radio waves to produce detailed images of the organs and tissues within the body. This non-invasive imaging technology is crucial for diagnosing a variety of conditions, from brain tumors to torn ligaments.
Principles of MRI
MRI is based on the principles of nuclear magnetic resonance (NMR), a technique that exploits the magnetic properties of certain atomic nuclei. Here’s how it works:
- Alignment of Nuclear Spins: he human body is largely made up of water molecules, which contain hydrogen atoms. In an MRI machine, a powerful magnetic field is used to align the magnetic moments of the hydrogen nuclei (protons) with the direction of the field.
- Radiofrequency (RF) Pulse Excitation: Once the protons are aligned, a series of radiofrequency pulses is applied. These pulses perturb the alignment of the protons away from the magnetic field.
- Relaxation and Signal Emission: After the RF pulse is turned off, the protons begin to return to their original alignment. As they relax, they emit signals, which are detected by the MRI scanner. The time it takes for the protons to relax provides important information about the chemical nature of the tissues.
- Image Construction: The signals emitted by the hydrogen atoms are processed to generate images that represent the structure and composition of body tissues. The brightness of each point in the image depends on the properties of the tissue, particularly its water content and how quickly the protons in the tissue return to their equilibrium state.
Examples
Example 1: Bar Magnets
A bar magnet is a classic example of a magnetic object. It has a north and south pole, and it produces a magnetic field around itself. When placed near iron filings, the filings align along the magnetic field lines, visually demonstrating the presence and direction of the magnetic field. Bar magnets are commonly used in various applications, including compasses, where they help determine direction.
Example 2: Electromagnets
Electromagnets are created by passing an electric current through a coil of wire, usually wrapped around a ferromagnetic core. This generates a magnetic field that can be turned on or off by controlling the current. Electromagnets are widely used in applications such as electric motors, magnetic locks, and industrial cranes to lift heavy metal objects, showcasing their utility in various fields.
Example 3: Magnetic Resonance Imaging (MRI)
MRI is a medical imaging technique that uses strong magnetic fields and radio waves to create detailed images of the body’s internal structures. The magnetic field aligns the protons in the body’s hydrogen atoms, and when the field is turned off, these protons emit signals that are captured to create images. This application of magnetism is crucial in diagnosing various medical conditions.
Example 4: Magnetic Fields of Earth
Earth itself behaves like a giant magnet, with a magnetic field that extends into space. This geomagnetic field is responsible for navigation using compasses, as it influences the orientation of magnetic needles. The Earth’s magnetic field protects the planet from solar winds and cosmic radiation, making it essential for maintaining life.
Example 5: Magnetic Levitation
Magnetic levitation (maglev) is a technology that uses magnets to lift and propel objects without physical contact. This principle is applied in maglev trains, which float above the tracks using powerful electromagnets. This reduction in friction allows for faster and smoother travel, demonstrating a practical application of magnetism in modern transportation systems.
Practice Questions
Question 1
What is the primary source of magnetism in materials?
A) Electric charge movement
B) Thermal energy
C) Gravitational forces
D) Chemical reactions
Correct Answer: A) Electric charge movement.
Explanation: Magnetism primarily arises from the movement of electric charges, particularly the electrons within atoms. When electrons move, such as when they orbit the nucleus or spin, they create magnetic fields. In certain materials, like ferromagnetic materials, the alignment of these atomic magnetic moments can lead to a net magnetic effect, making them strong magnets.
Question 2
Which of the following materials is considered a ferromagnet?
A) Copper
B) Aluminum
C) Iron
D) Gold
Correct Answer: C) Iron.
Explanation: Ferromagnetic materials are those that can be magnetized and maintain their magnetic properties after an external magnetic field is removed. Iron is the most common example of a ferromagnetic material, exhibiting strong magnetic properties. In contrast, copper and aluminum are non-magnetic, while gold is a diamagnetic material that does not exhibit permanent magnetism.
Question 3
What happens to the magnetic field of a solenoid when the current flowing through it is increased?
A) The magnetic field decreases.
B) The magnetic field becomes weaker.
C) The magnetic field remains unchanged.
D) The magnetic field becomes stronger.
Correct Answer: D) The magnetic field becomes stronger.
Explanation: A solenoid is a coil of wire that generates a magnetic field when an electric current passes through it. According to the principles of electromagnetism, increasing the current flowing through the solenoid enhances the strength of the magnetic field it produces. This is because the magnetic field strength is directly proportional to the amount of current flowing through the wire, leading to a stronger magnetic effect as the current increases.