Preparing for the MCAT requires a strong grasp of bioenergetics, a core concept in biochemistry. This topic covers energy transformation within cells, focusing on ATP production, thermodynamics, and metabolic pathways like glycolysis and oxidative phosphorylation. Understanding how cells harness and utilize energy is essential for mastering topics related to metabolism, enzyme function, and cellular regulation. A thorough knowledge of bioenergetics is critical for achieving a high score on the MCAT, as it underpins many biological processes.
Learning Objectives
When studying Principles of Bioenergetics for the MCAT, your learning objectives should include understanding how cells produce, store, and utilize energy through metabolic pathways. You should focus on mastering concepts of thermodynamics, ATP hydrolysis, and energy coupling in cellular reactions. Additionally, learn the details of glycolysis, the citric acid cycle, and oxidative phosphorylation, emphasizing their roles in energy production. Grasping enzyme regulation, energy yield calculations, and the impact of factors like pH and temperature on these processes is essential for the MCAT.
Key Concepts in Bioenergetics
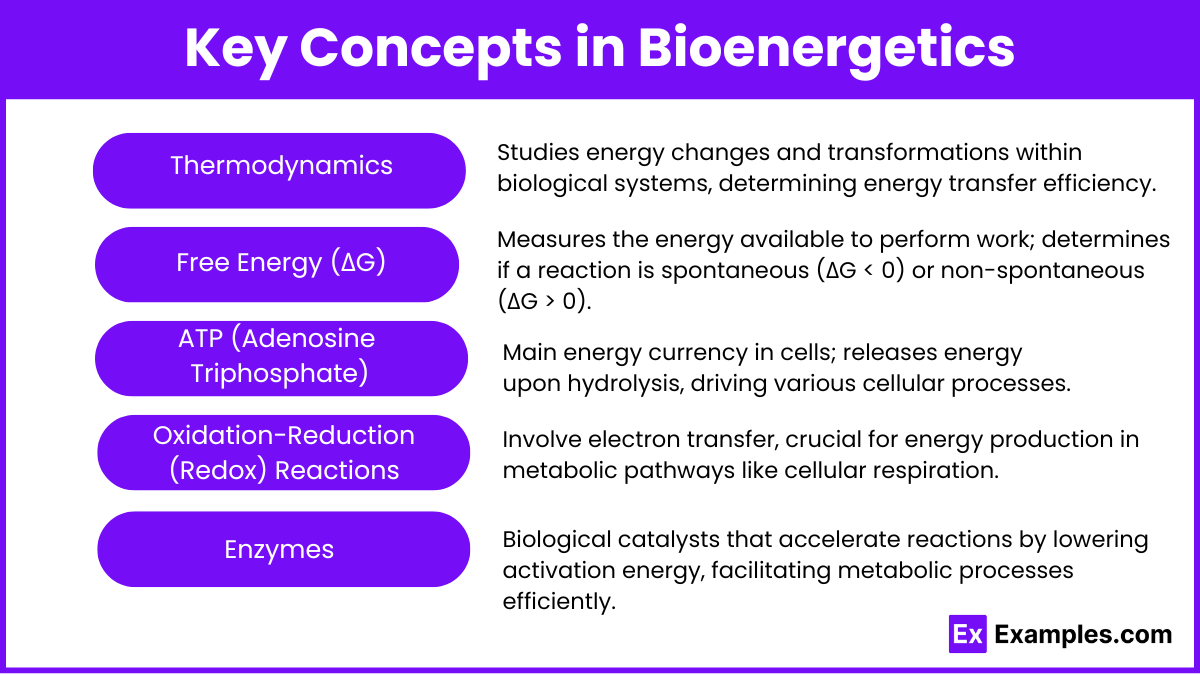
- Thermodynamics:
- First Law: Energy cannot be created or destroyed; it can only be transformed. In cells, this transformation occurs through various metabolic reactions that convert chemical energy from nutrients into ATP.
- Second Law: Energy transformations increase the entropy (disorder) of a system. In bioenergetics, this principle is evident as some energy is always lost as heat during metabolic processes.
- Free Energy (ΔG):
- The change in free energy (ΔG) of a reaction determines whether a reaction will proceed spontaneously.
- Exergonic Reactions (ΔG < 0): Release energy, driving processes like ATP synthesis and oxidative phosphorylation.
- Endergonic Reactions (ΔG > 0): Require an input of energy, as seen in biosynthesis and active transport across membranes.
- Equilibrium (ΔG = 0): Represents a state of no net change in energy, where forward and reverse reactions occur at equal rates.
- ATP (Adenosine Triphosphate):
- ATP consists of adenine, ribose, and three phosphate groups. The energy is stored in the bonds between the phosphate groups, particularly in the terminal phosphate bond.
- ATP Hydrolysis: When ATP is hydrolyzed to ADP and inorganic phosphate (Pi), it releases energy that can drive endergonic reactions, such as muscle contraction or active transport.
- Oxidation-Reduction (Redox) Reactions:
- In biological systems, redox reactions are vital for the transfer of electrons and energy.
- Oxidation: Loss of electrons, which usually occurs in catabolic pathways (e.g., the conversion of glucose to pyruvate).
- Reduction: Gain of electrons, often occurring in anabolic pathways (e.g., the synthesis of fatty acids from acetyl-CoA).
- NADH and FADH₂ are reduced forms of energy carriers that transport electrons to the electron transport chain for ATP production.
- Enzymes:
- Enzymes are biological catalysts that speed up reactions by lowering the activation energy.
- They are essential in bioenergetics because they facilitate key reactions in metabolic pathways, such as glycolysis and the Krebs cycle, ensuring that energy production occurs rapidly and efficiently.
- Enzymes are highly specific to substrates, meaning they ensure the correct metabolic reactions proceed, maintaining efficient energy transformation.
Types of Bioenergetic Pathways
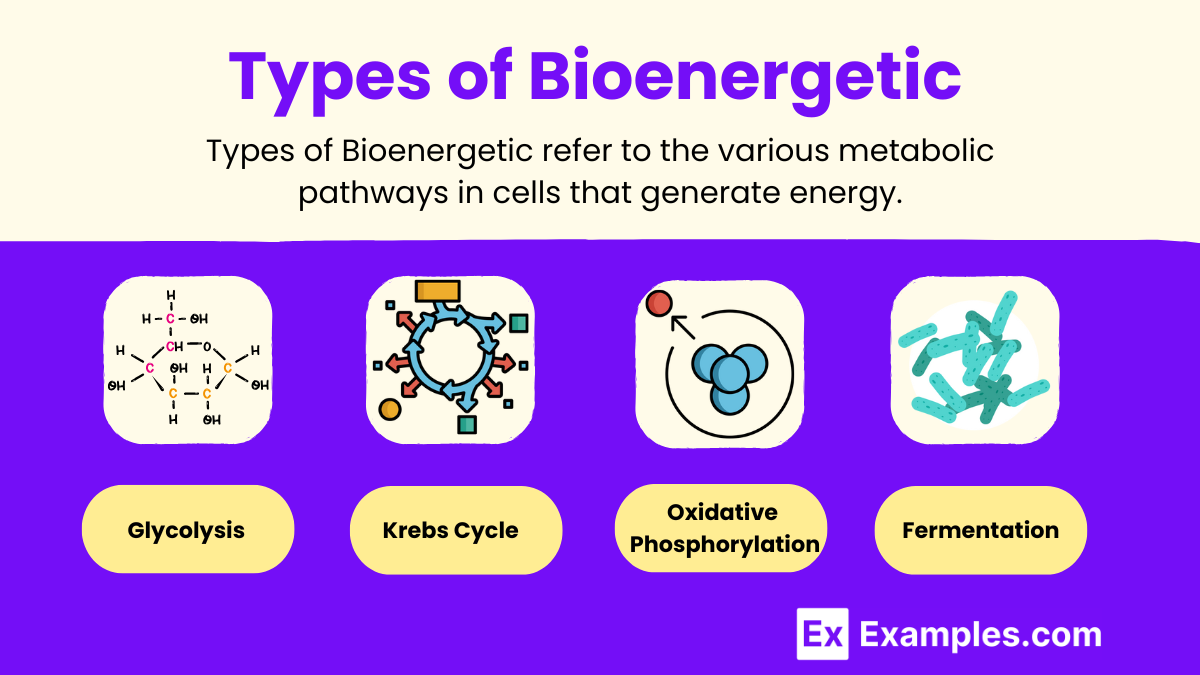
- Glycolysis:
- Occurs in the cytoplasm and does not require oxygen (anaerobic).
- It breaks down one glucose molecule into two pyruvate molecules, producing 2 ATP and 2 NADH.
- Glycolysis is the first step in both aerobic and anaerobic respiration, providing a quick source of ATP.
- Krebs Cycle (Citric Acid Cycle):
- Takes place in the mitochondrial matrix and requires oxygen (aerobic).
- It further oxidizes pyruvate into carbon dioxide, generating high-energy electron carriers: 3 NADH, 1 FADH₂, and 1 ATP per cycle.
- These carriers transport electrons to the electron transport chain, driving ATP production.
- Oxidative Phosphorylation:
- The final and most ATP-generating step of cellular respiration, occurring in the inner mitochondrial membrane.
- It relies on the electron transport chain, where electrons from NADH and FADH₂ move through a series of protein complexes, creating a proton gradient.
- The energy from this gradient is used by ATP synthase to produce ATP, with oxygen serving as the final electron acceptor.
- Fermentation:
- Occurs when oxygen is not available, allowing cells to produce energy anaerobically.
- In animals, fermentation results in the production of lactic acid, while in yeast, it results in ethanol and carbon dioxide.
- Although it produces less ATP compared to aerobic processes, it ensures ATP production can continue in anaerobic conditions.
Purpose of Bioenergetics in Biological Systems
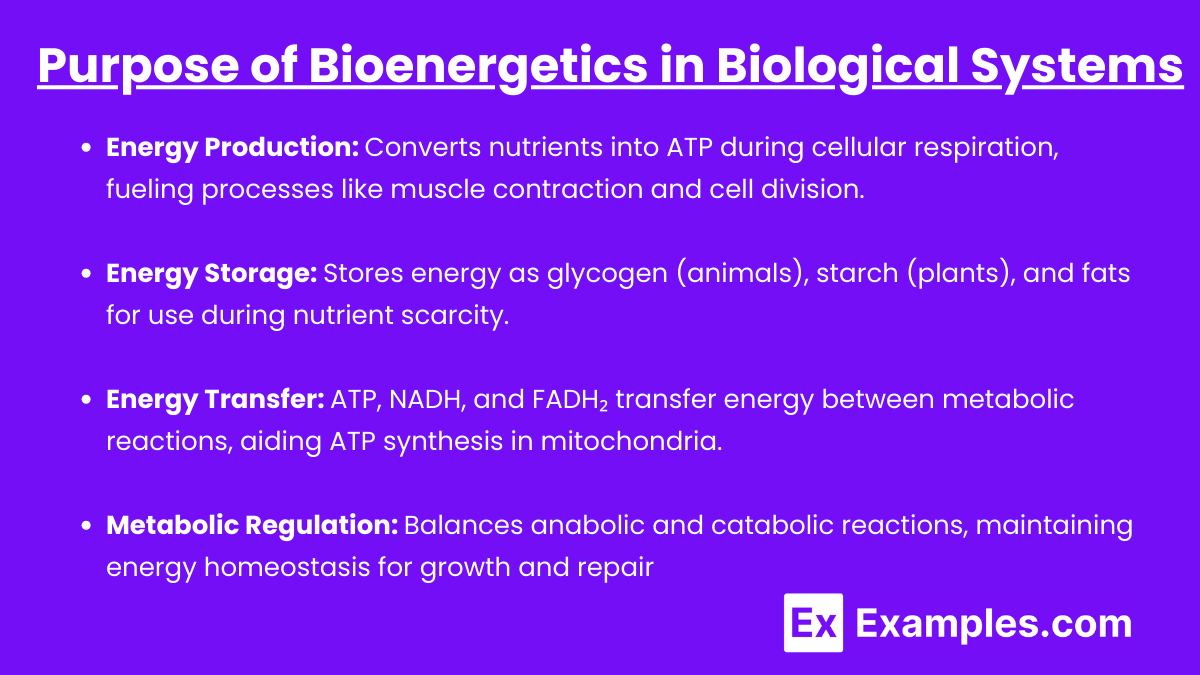
- Energy Production:
- Bioenergetics is central to energy production, allowing cells to convert nutrients into usable energy, primarily in the form of ATP.
- For example, during cellular respiration, glucose is broken down in a series of metabolic reactions to release energy stored in chemical bonds.
- This energy is then used to fuel cellular processes such as muscle contraction, protein synthesis, and cell division.
- Energy Storage:
- Energy is not only produced but also stored for later use. Glycogen (in animals) and starch (in plants) are common energy storage forms.
- Fat storage (triglycerides) also serves as a long-term energy reserve, which is crucial for sustaining cellular functions during periods of nutrient scarcity.
- Energy Transfer:
- Molecules like ATP, NADH, and FADH₂ act as energy carriers, facilitating the transfer of energy between catabolic (breakdown) and anabolic (synthesis) reactions.
- For instance, NADH and FADH₂ transport electrons to the electron transport chain, enabling the production of ATP in mitochondria.
- Metabolic Regulation:
- Bioenergetics helps regulate metabolism by adjusting anabolic and catabolic reactions to meet cellular energy demands.
- This regulation ensures homeostasis, maintaining an optimal balance of energy for growth, repair, and other vital functions.
Examples
Example 1. Cellular Respiration in Muscle Contraction:
During intense physical activity, muscle cells rely on bioenergetic principles to generate the necessary energy for contraction. Glycolysis rapidly converts glucose to pyruvate, providing ATP for immediate energy. As the exercise continues, the Krebs cycle and oxidative phosphorylation increase ATP production to sustain prolonged activity. This efficient energy conversion, coupled with ATP hydrolysis, powers the actin-myosin interaction in muscle fibers, enabling contraction and relaxation.
Example 2. Maintaining Blood Glucose Levels through Gluconeogenesis:
The liver uses the principles of bioenergetics to regulate blood glucose levels, particularly during fasting or strenuous exercise. When glucose levels drop, the liver initiates gluconeogenesis, a process that requires ATP to synthesize glucose from non-carbohydrate sources like amino acids and lactate. By using energy stored in ATP and other energy carriers, gluconeogenesis helps maintain a stable glucose supply for tissues like the brain and red blood cells, which depend heavily on glucose for energy.
Example 3. Fatty Acid Oxidation in Adipose Tissue:
Adipose tissue stores energy in the form of triglycerides, which are broken down into fatty acids during energy scarcity. These fatty acids undergo beta-oxidation, a series of redox reactions that convert them into acetyl-CoA, entering the Krebs cycle. Oxidative phosphorylation then produces large amounts of ATP from the electrons carried by NADH and FADH₂, ensuring an efficient energy supply for essential cellular functions during fasting or prolonged exercise.
Example 4. Energy Production in Red Blood Cells through Anaerobic Glycolysis:
Red blood cells lack mitochondria and rely solely on anaerobic glycolysis to meet their energy needs. This metabolic pathway uses the principles of bioenergetics to break down glucose into pyruvate, yielding 2 ATP molecules per glucose molecule without requiring oxygen. Despite the lower energy output compared to aerobic processes, this pathway ensures a continuous ATP supply, enabling red blood cells to maintain their shape, ion gradients, and transport oxygen effectively throughout the body.
Example 5. Proton Motive Force in ATP Synthesis:
In mitochondria, the electron transport chain (ETC) uses redox reactions to pump protons across the inner membrane, creating a proton gradient, also known as the proton motive force. This gradient represents stored energy, which ATP synthase harnesses to convert ADP into ATP. The efficient conversion of the proton motive force into ATP demonstrates the core principle of energy coupling, where the energy from electron transfer is used directly to synthesize ATP, illustrating the link between redox reactions and energy production.
Practice Questions
Question 1
Which of the following statements about ATP is true in the context of bioenergetics?
A) ATP synthesis is an endergonic process that requires energy input.
B) ATP stores energy in its adenine base.
C) ATP releases energy when hydrolyzed to ADP and inorganic phosphate.
D) ATP can only be generated anaerobically.
Correct Answer: C) ATP releases energy when hydrolyzed to ADP and inorganic phosphate.
Explanation:
- ATP is the primary energy currency of cells, storing energy in the high-energy phosphate bonds.
- Statement C is true because when ATP is hydrolyzed to ADP and inorganic phosphate (Pi), energy is released, making it available to drive cellular reactions (e.g., muscle contraction, active transport).
- Statement A is incorrect because ATP synthesis from ADP and Pi during processes like oxidative phosphorylation is an exergonic process that is coupled to energy-releasing reactions.
- Statement B is incorrect because ATP stores energy primarily in its phosphate bonds, not in the adenine base.
- Statement D is incorrect as ATP can be generated both anaerobically (e.g., glycolysis) and aerobically (e.g., oxidative phosphorylation).
Question 2
Which of the following pathways generates the most ATP during glucose metabolism?
A) Glycolysis
B) Krebs Cycle
C) Oxidative Phosphorylation
D) Fermentation
Correct Answer: C) Oxidative Phosphorylation
Explanation:
- Oxidative phosphorylation is the process where most ATP is generated during glucose metabolism. It occurs in the inner mitochondrial membrane and involves the electron transport chain (ETC) and ATP synthase. It produces around 26-28 ATP molecules per glucose molecule, making it the most ATP-productive pathway.
- Glycolysis (Option A) generates only 2 ATP molecules directly.
- Krebs Cycle (Option B) produces 2 ATP molecules directly, but it generates high-energy electron carriers (NADH and FADH₂) that fuel oxidative phosphorylation.
- Fermentation (Option D), an anaerobic process, produces only 2 ATP molecules per glucose molecule and is far less efficient than oxidative phosphorylation.
Question 3
What is the primary role of NADH and FADH₂ in bioenergetics?
A) They act as direct sources of ATP in the cytoplasm.
B) They provide electrons for oxidative phosphorylation.
C) They store glucose for later energy use.
D) They function as enzymes in the Krebs Cycle.
Correct Answer: B) They provide electrons for oxidative phosphorylation.
Explanation:
- NADH and FADH₂ are essential energy carriers in bioenergetics. They are produced during glycolysis, the Krebs cycle, and beta-oxidation, and their primary role is to donate electrons to the electron transport chain (ETC) during oxidative phosphorylation.
- Option A is incorrect because NADH and FADH₂ are not direct sources of ATP; they contribute to ATP synthesis indirectly by donating electrons to the ETC.
- Option C is incorrect as they do not store glucose but instead store and transfer energy in the form of electrons.
- Option D is incorrect because NADH and FADH₂ are not enzymes; they are coenzymes and electron carriers that play a role in multiple metabolic reactions, including the Krebs cycle.