Proton Nuclear Magnetic Resonance (¹H NMR) is essential for understanding molecular structure and dynamics, playing a pivotal role in analytical chemistry and biochemistry. On the MCAT, you’ll explore proton environments, chemical shifts, coupling constants, and spin-spin splitting patterns. Mastering ¹H NMR will allow you to interpret spectra and determine molecular structures accurately. Understanding shielding, deshielding, and peak integration is critical, as these concepts are vital for excelling in both the chemistry and organic chemistry sections of the exam.
Learning Objectives
In studying “Proton Nuclear Magnetic Resonance (1H-NMR)” for the MCAT, you should learn to interpret NMR spectra by identifying the number, position, and intensity of peaks to determine molecular structure. Understand the concept of chemical shift and how electron shielding/deshielding affects peak positions. Recognize how spin-spin coupling creates splitting patterns (multiplets) and use the n+1 rule to predict the number of neighboring protons. Evaluate integration values to determine the relative number of equivalent protons contributing to each signal. Practice correlating NMR spectra with molecular fragments to elucidate structures. Additionally, develop proficiency in distinguishing between equivalent and non-equivalent protons to solve organic chemistry problems relevant to MCAT passages.
Interpreting NMR Spectra: Peaks, Positions, and Intensities
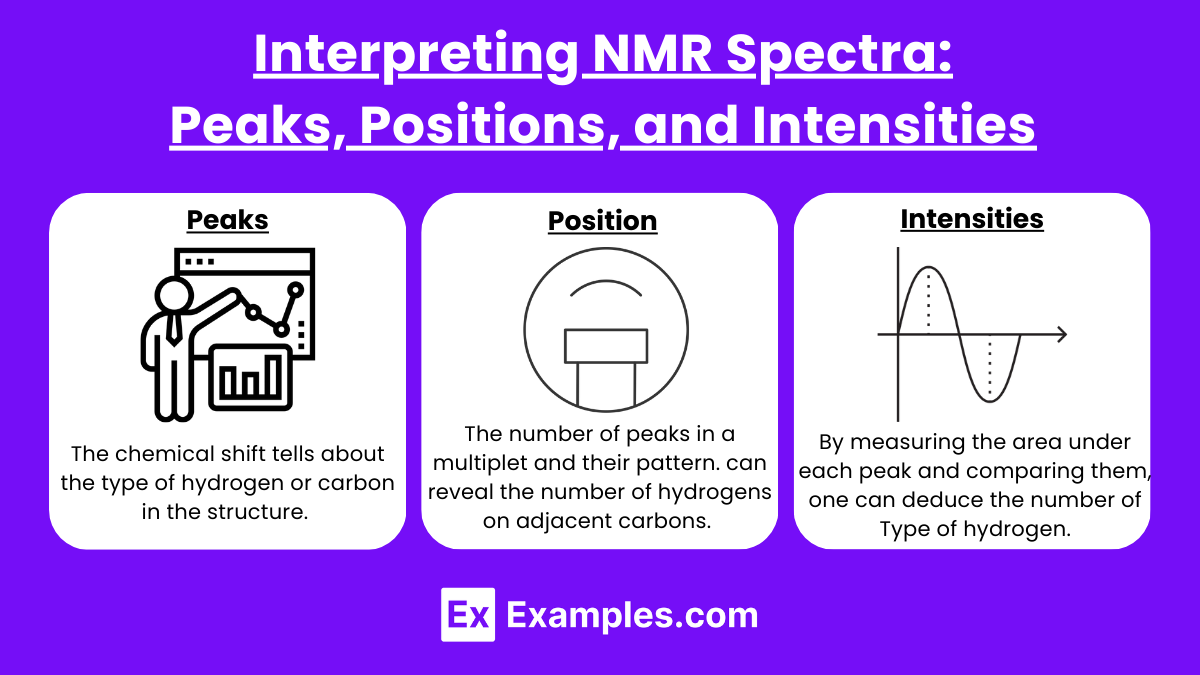
Nuclear Magnetic Resonance (NMR) spectroscopy is a sophisticated analytical tool used primarily to determine the structure of organic compounds. It is based on the absorption of radiofrequency radiation by nuclei in a magnetic field, leading to transitions between magnetic energy levels. The information obtained from NMR spectra includes the chemical environment of the nuclei, their interactions, and molecular dynamics.
Fundamentals of NMR Spectroscopy
- Nuclei Observed: The most commonly studied nuclei in NMR are 1H (proton NMR) and 13C, although other isotopes like 19F and 31P can also be analyzed.
- Magnetic Field: Nuclei with odd mass or atomic numbers have a property called spin. When placed in a magnetic field, these spins align either with or against the field, creating different energy states.
- Radiofrequency Pulse: When a radiofrequency pulse is applied, it causes nuclei in the lower energy state to absorb energy and flip to the higher energy state. When the pulse is turned off, the nuclei relax back to the lower energy state, emitting radiofrequency signals that are detected and transformed into an NMR spectrum.
Interpreting Peaks, Positions, and Intensities
- Peaks and Chemical Shift: The chemical shift tells about the type of hydrogen or carbon in the structure. For instance, in 1H NMR, a peak around 0-3 ppm usually indicates aliphatic hydrogens, 4-6 ppm indicates hydrogens on a carbon bonded to an electronegative atom, and 6-8 ppm generally indicates aromatic hydrogens.
- Splitting Patterns: The number of peaks in a multiplet and their pattern (doublet, triplet, quartet, etc.) can reveal the number of hydrogens on adjacent carbons. The N+1 rule often helps predict this pattern, where N is the number of neighboring equivalent hydrogens.
- Integration: By measuring the area under each peak and comparing them, one can deduce the relative number of each type of hydrogen. This is critical for determining molecular structures, especially in complex organic compounds.
- Examples:
- A triplet at around 1 ppm often indicates a methyl group (−CH3) adjacent to a methylene group (−CH2−).
- A quartet around 4 ppm usually suggests a methylene group (−CH2−) next to an electronegative atom or a carbonyl group, especially in environments like esters or ethers.
Concept of Chemical Shift and Electron Shielding/Deshielding
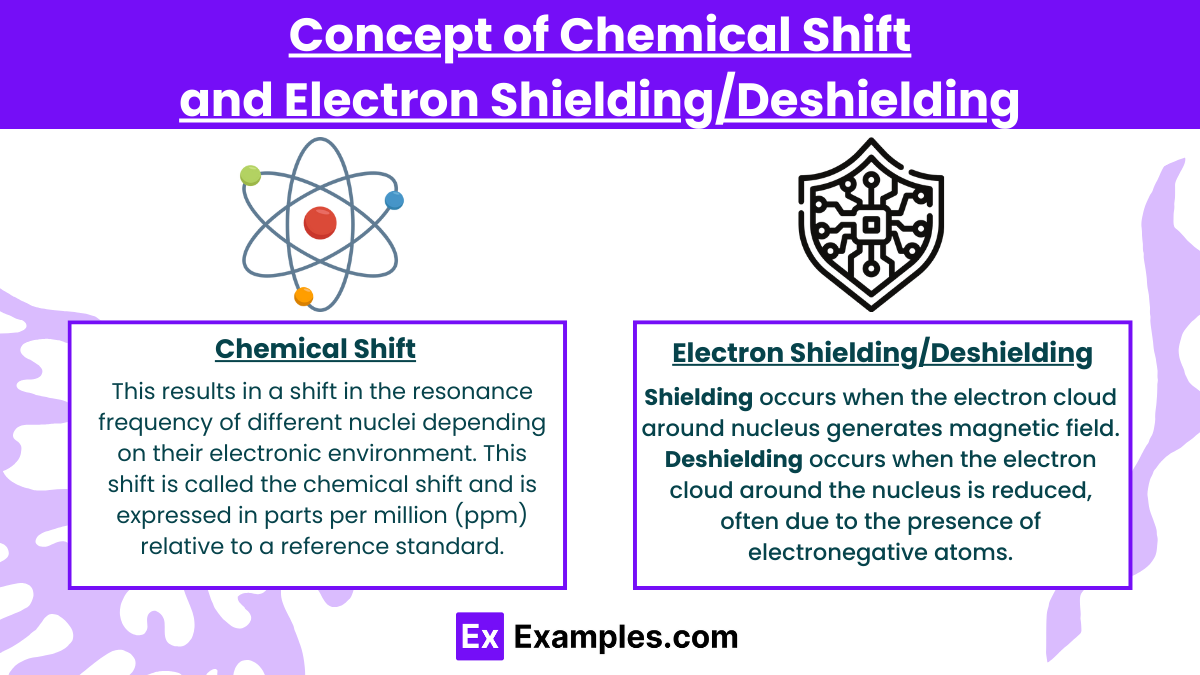
The concept of chemical shift in Nuclear Magnetic Resonance (NMR) spectroscopy refers to the variation in the resonant frequency of a nucleus due to its local electronic environment. The chemical shift provides critical information about the structure and electronic properties of a molecule by indicating the relative position of NMR signals on the spectrum.
Chemical Shift
In NMR spectroscopy, nuclei (typically ¹H or 13C) are placed in a strong external magnetic field, causing them to align either with or against the field. However, the effective magnetic field experienced by each nucleus is not solely the external field; it is also influenced by the electron density around the nucleus. This results in a shift in the resonance frequency of different nuclei depending on their electronic environment. This shift is called the chemical shift and is expressed in parts per million (ppm) relative to a reference standard, typically tetramethylsilane (TMS) for 1H and 13C NMR.
Electron Shielding and Deshielding
The chemical shift arises because electrons around the nucleus generate small magnetic fields that either oppose or reinforce the external magnetic field. This phenomenon is referred to as shielding or deshielding, depending on whether the local electron density increases or decreases the effective magnetic field experienced by the nucleus.
Shielding
- Shielding occurs when the electron cloud around the nucleus generates a magnetic field that opposes the external magnetic field. As a result, the nucleus experiences a reduced effective magnetic field, and its resonant frequency is lower than it would be without the shielding effect.
- Result in the NMR Spectrum: Shielded nuclei appear upfield, or at lower chemical shifts (toward the right side of the spectrum). For example, alkane protons, which are surrounded by electron-dense environments, typically resonate around 0.5–2 ppm in 1H NMR.
Deshielding
- Deshielding occurs when the electron cloud around the nucleus is reduced, often due to the presence of electronegative atoms or other groups that withdraw electron density from the nucleus. This decreases the opposing magnetic field from the electrons, allowing the nucleus to experience a stronger effective magnetic field.
- Result in the NMR Spectrum: Deshielded nuclei resonate downfield, or at higher chemical shifts (toward the left side of the spectrum). For example, protons near electronegative atoms such as oxygen or halogens in alcohols, esters, or acids are deshielded and typically resonate at 3–5 ppm or higher.
Factors Affecting Chemical Shift
- Electronegativity of Nearby Atoms: Electronegative atoms (like oxygen, nitrogen, or halogens) withdraw electron density, deshielding nearby protons or carbons and causing downfield shifts. For instance, the proton in a hydroxyl group (-OH) typically appears further downfield compared to a methyl group (-CH₃).
- Hybridization of Carbon Atoms: The hybridization state of carbon atoms affects the electron density around hydrogen atoms. Protons attached to sp² hybridized carbons, such as in alkenes or aromatic rings, are more deshielded than those attached to sp³ carbons in alkanes.
- Aromaticity: Aromatic compounds exhibit ring current effects, where circulating π-electrons generate strong magnetic fields that deshield protons located outside the ring plane, resulting in significant downfield shifts (often 6–8 ppm in benzene-like structures).
- Hydrogen Bonding: Hydrogen bonding can significantly deshield protons, especially in alcohols, amines, or carboxylic acids, shifting them downfield.
Evaluating Integration Values for Equivalent Protons
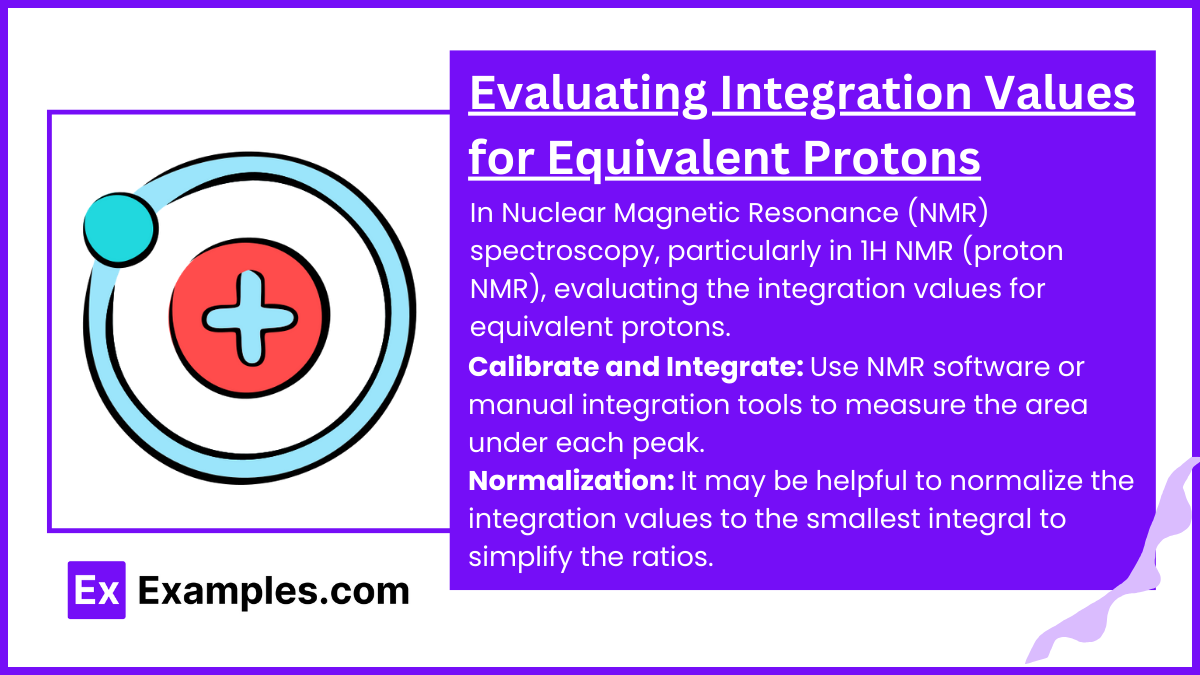
In Nuclear Magnetic Resonance (NMR) spectroscopy, particularly in 1H NMR (proton NMR), evaluating the integration values for equivalent protons is a crucial step for determining the structure of a molecule. Integration of the NMR signals provides a quantitative measure of the number of protons that contribute to each signal, which reflects the number of chemically equivalent protons in the molecule.
Understanding Integration in 1H NMR
Integration refers to the process of measuring the area under the peaks in an NMR spectrum. This area is proportional to the number of nuclei (protons, in the case of 1H NMR) causing the signal. Here’s how integration values aid in structural analysis:
- Equivalent Protons: Protons in the same chemical environment (i.e., chemically equivalent) will produce a single NMR signal. The area under this signal, when measured relative to other signals, tells you how many protons contribute to that environment.
- Proportional Representation: The integral of a peak relative to others in the spectrum provides a direct ratio of the number of equivalent protons associated with each signal.
Steps for Evaluating Integration Values
- Record the NMR Spectrum: Obtain the 1H NMR spectrum of the compound. Make sure that the spectrum is well-resolved with clear separation of signals.
- Calibrate and Integrate: Use NMR software or manual integration tools to measure the area under each peak. Modern NMR spectrometers often provide digital integration tools that automatically calculate and display these areas as numerical values or as graphical overlays on the peaks.
- Compare Integrations: Compare the integration values of different signals to determine the ratio of equivalent protons contributing to each signal. For example, if one signal integrates to a value of 3 and another to 1, it suggests that there are three times as many protons contributing to the first signal as to the second.
- Normalization: It may be helpful to normalize the integration values to the smallest integral to simplify the ratios. For example, if the smallest integral in a spectrum is 0.5 and another peak has an integral of 1.5, normalizing these would give you relative integrals of 1 and 3, respectively.
Distinguishing Between Equivalent and Non-Equivalent Protons
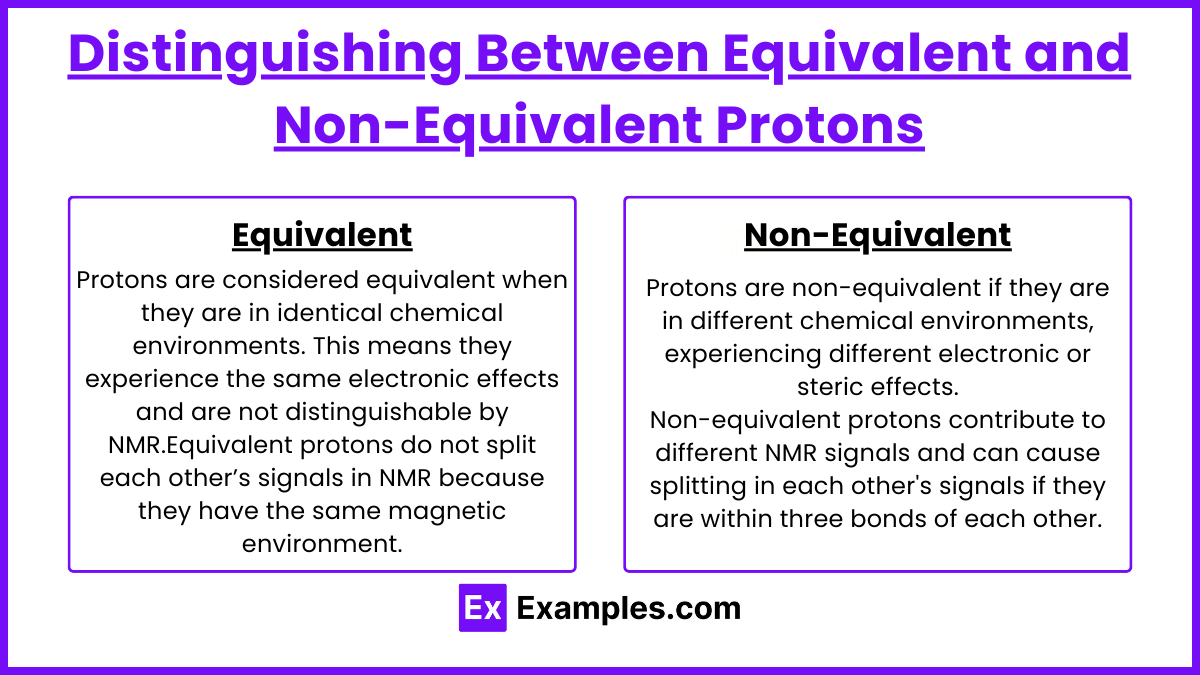
Distinguishing between equivalent and non-equivalent protons is crucial in 1H NMR (proton NMR) spectroscopy as it provides insights into the symmetry and environment of various hydrogen atoms within a molecule. Understanding whether protons are equivalent or non-equivalent helps in interpreting the NMR spectra accurately, which is essential for structural elucidation.
What Makes Protons Equivalent or Non-Equivalent?
Equivalent Protons:
- Protons are considered equivalent when they are in identical chemical environments. This means they experience the same electronic effects and are not distinguishable by NMR.
- Equivalent protons do not split each other’s signals in NMR because they have the same magnetic environment.
Non-Equivalent Protons:
- Protons are non-equivalent if they are in different chemical environments, experiencing different electronic or steric effects.
- Non-equivalent protons contribute to different NMR signals and can cause splitting in each other’s signals if they are within three bonds of each other.
How to Determine Equivalence?
- Symmetry: Analyze the symmetry of the molecule. Protons in symmetric positions, such as those on a symmetrically substituted benzene ring or on methyl groups in a symmetric environment, are typically equivalent.
- Chemical Environment: Consider the groups or atoms attached to the carbons bearing the protons. Different groups will cause different shifts and splitting patterns, making the protons non-equivalent.
- NMR Spectrum Analysis:
- Signal Count: The number of signals in a 1H NMR spectrum generally corresponds to the number of sets of equivalent protons. Fewer signals than the number of proton types indicate the presence of equivalent protons.
- Splitting Patterns: Examine the multiplicity of each signal. Equivalent protons do not split each other’s signals, but they do contribute to the intensity (integral) of the signal.
Practical Steps in Spectrum Analysis
- Identify Signals: First, identify and count distinct signals in the NMR spectrum. Each signal corresponds to a set of equivalent protons.
- Analyze Splitting Patterns: Look at the multiplet structure (singlet, doublet, triplet, etc.) of each signal to determine interactions with adjacent, non-equivalent protons.
- Compare Chemical Shifts: Shifts can provide clues about the electronic environment of protons. For example, protons near electronegative groups appear downfield (at higher ppm).
Examples
Example 1: Structural Elucidation of Organic Compounds
Proton NMR spectroscopy is widely used in organic chemistry to determine the structure of unknown compounds. For instance, when analyzing a sample of an organic molecule, such as an alcohol or a ketone, chemists can use the NMR spectrum to identify the types of hydrogen environments present. The number of peaks, their positions, and the splitting patterns provide valuable information regarding the molecular structure, including the connectivity of atoms and the presence of functional groups.
Example 2: Studying Chemical Reactions
Proton NMR can be used to monitor the progress of chemical reactions in real time. For example, in a reaction where an alcohol is converted to an alkene, chemists can take NMR measurements at various time intervals to observe changes in the hydrogen signals. This allows them to determine the reaction kinetics and confirm the formation of the desired product by analyzing the disappearance of the alcohol signal and the emergence of the alkene signal.
Example 3: Identifying Isomers
NMR spectroscopy is effective in distinguishing between structural isomers and stereoisomers. For example, in the case of butanol, the proton NMR spectrum can reveal differences in chemical shifts based on the hydrogen environments around the carbon atoms. By comparing the spectra of different butanol isomers, chemists can identify the specific isomer present in a sample, providing insights into its structural characteristics.
Example 4: Analysis of Complex Mixtures
Proton NMR is particularly useful for analyzing complex mixtures, such as natural products or biological samples. For instance, in the analysis of essential oils, proton NMR can identify various components within the mixture based on their unique hydrogen environments. This technique allows researchers to determine the composition of the essential oil and assess its potential therapeutic properties, making it a valuable tool in pharmacognosy and natural product chemistry.
Example 5: Biomolecular Studies
Proton NMR is instrumental in studying biomolecules, such as proteins and nucleic acids. In structural biology, NMR spectroscopy can provide information about the three-dimensional structure of proteins in solution. For example, researchers can analyze the hydrogen bonding patterns and interactions within a protein to understand its folding and stability. This application of NMR is crucial for drug design and understanding biological processes at the molecular level.
Practice Questions
Question 1
What is the primary purpose of proton nuclear magnetic resonance (NMR) spectroscopy?
A) To measure the mass of molecules
B) To identify the structure of organic compounds
C) To determine the concentration of solutions
D) To visualize the spatial arrangement of atoms
Correct Answer: B) To identify the structure of organic compounds.
Explanation: Proton NMR spectroscopy is primarily used to determine the structure of organic compounds. It provides detailed information about the hydrogen atoms in a molecule, including their environment, connectivity, and the presence of functional groups. By analyzing the NMR spectrum, chemists can deduce the molecular structure, which is essential for organic chemistry and material science. Options A and C pertain to other analytical techniques, while D, though partially true, is more relevant to techniques like X-ray crystallography.
Question 2
Which of the following factors can affect the chemical shift observed in a proton NMR spectrum?
A) The magnetic field strength used in the NMR machine
B) The temperature of the sample
C) The electronic environment surrounding the hydrogen atoms
D) All of the above
Correct Answer: D) All of the above.
Explanation: The chemical shift in proton NMR is influenced by several factors, including the magnetic field strength, the temperature of the sample, and the electronic environment surrounding the hydrogen atoms. Variations in the electronic environment cause different hydrogen atoms to experience different magnetic fields, resulting in shifts in their resonance frequency. Thus, all these factors contribute to the observed chemical shifts, making option D the correct answer.
Question 3
In proton NMR, what does the term “integration” refer to?
A) The measurement of the sample temperature
B) The process of increasing the magnetic field strength
C) The area under the peaks in the NMR spectrum
D) The adjustment of chemical shifts
Correct Answer: C) The area under the peaks in the NMR spectrum.
Explanation: In proton NMR spectroscopy, integration refers to the measurement of the area under each peak in the NMR spectrum. This area is directly proportional to the number of protons contributing to that signal. Therefore, integration provides information about the relative number of hydrogen atoms in different environments within the molecule, allowing chemists to deduce how many hydrogen atoms are associated with specific groups or functional groups. Options A and B do not relate to integration, and option D refers to chemical shifts, not integration.