Sound, a key concept in the MCAT, explores wave properties, including frequency, wavelength, and amplitude. Topics include sound propagation, Doppler effect, resonance, decibel levels, and how sound waves interact with different media, such as solids, liquids, and gases, impacting hearing and medical applications like ultrasounds.
Learning Objectives
In studying “Sound” for the MCAT, you should learn to understand the nature of sound as a mechanical wave that requires a medium for propagation. Differentiate between the key properties of sound waves, including frequency, wavelength, amplitude, and speed, and how they relate to pitch and intensity. Understand the principles of wave interference, resonance, and standing waves. Analyze the Doppler effect and its applications in real-world scenarios. Evaluate the relationship between sound intensity, decibels, and perception of loudness. Study how sound travels through different media and how factors like temperature and pressure affect its speed. Additionally, gain proficiency in understanding the role of sound in biological systems, such as hearing mechanisms in the human ear and medical applications like ultrasound.
Understanding the Nature of Sound as a Mechanical Wave
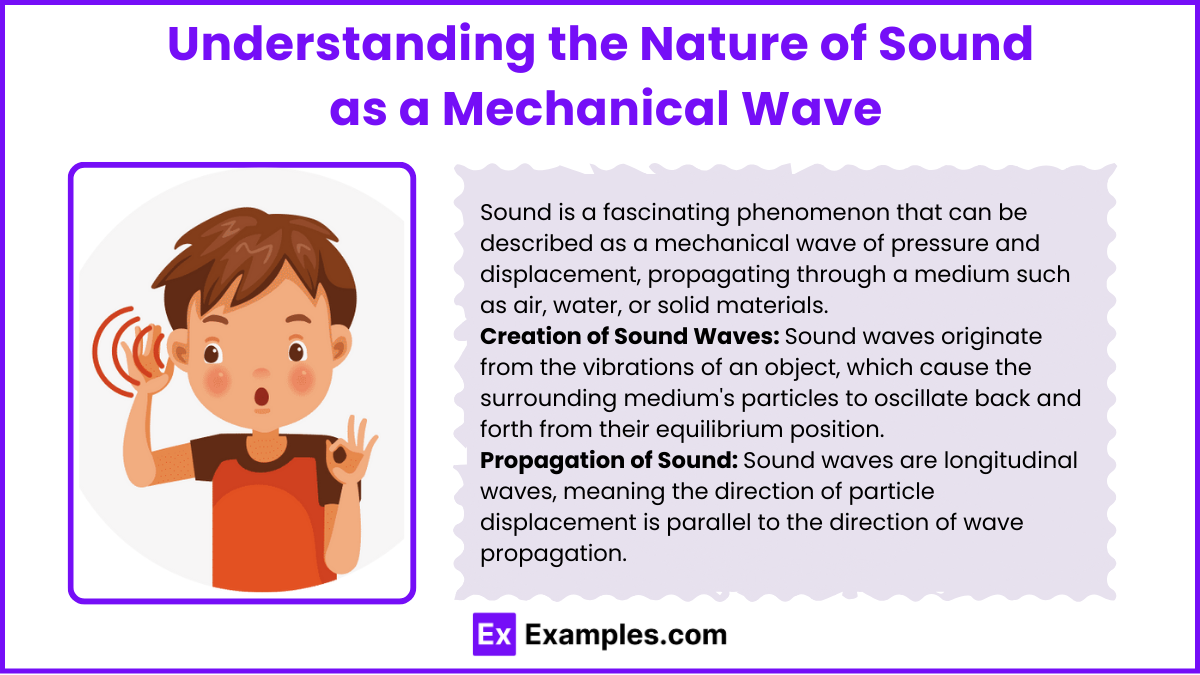
Sound is a fascinating phenomenon that can be described as a mechanical wave of pressure and displacement, propagating through a medium such as air, water, or solid materials. Unlike electromagnetic waves like light, sound requires a medium to travel through and cannot propagate through a vacuum. Understanding the nature of sound as a mechanical wave involves exploring its creation, propagation, and characteristics.
Creation of Sound Waves
Sound waves originate from the vibrations of an object, which cause the surrounding medium’s particles to oscillate back and forth from their equilibrium position. This oscillation creates regions of compression and rarefaction:
- Compression: Areas where particles are pushed together, increasing the pressure.
- Rarefaction: Areas where particles are pulled apart, decreasing the pressure.
This alternating pattern of compression and rarefaction moves away from the source as a wave.
Propagation of Sound
Sound waves are longitudinal waves, meaning the direction of particle displacement is parallel to the direction of wave propagation. Here’s how sound travels through various media:
- In Air: Sound travels as a series of compressions and rarefactions of air molecules.
- In Liquids and Solids: Sound can also propagate efficiently through liquids and solids due to their denser molecular structure, often at higher speeds than in air because the particles are closer together and interact more strongly.
Characteristics of Sound Waves
Sound waves have several key characteristics that define their perception and physical properties:
- Frequency: The number of pressure oscillations per second, measured in hertz (Hz). Frequency determines the pitch of the sound; higher frequencies are perceived as higher pitches.
- Wavelength: The distance over which the wave’s shape repeats. It is inversely proportional to frequency, meaning higher frequencies have shorter wavelengths.
- Amplitude: The height of the wave, which corresponds to the volume or loudness of the sound. Larger amplitudes are perceived as louder sounds.
- Speed: The speed of sound varies depending on the medium. In air at room temperature, it travels at approximately 343 meters per second (1,125 ft/s). Sound travels faster in liquids and even faster in solids.
Key Properties of Sound Waves
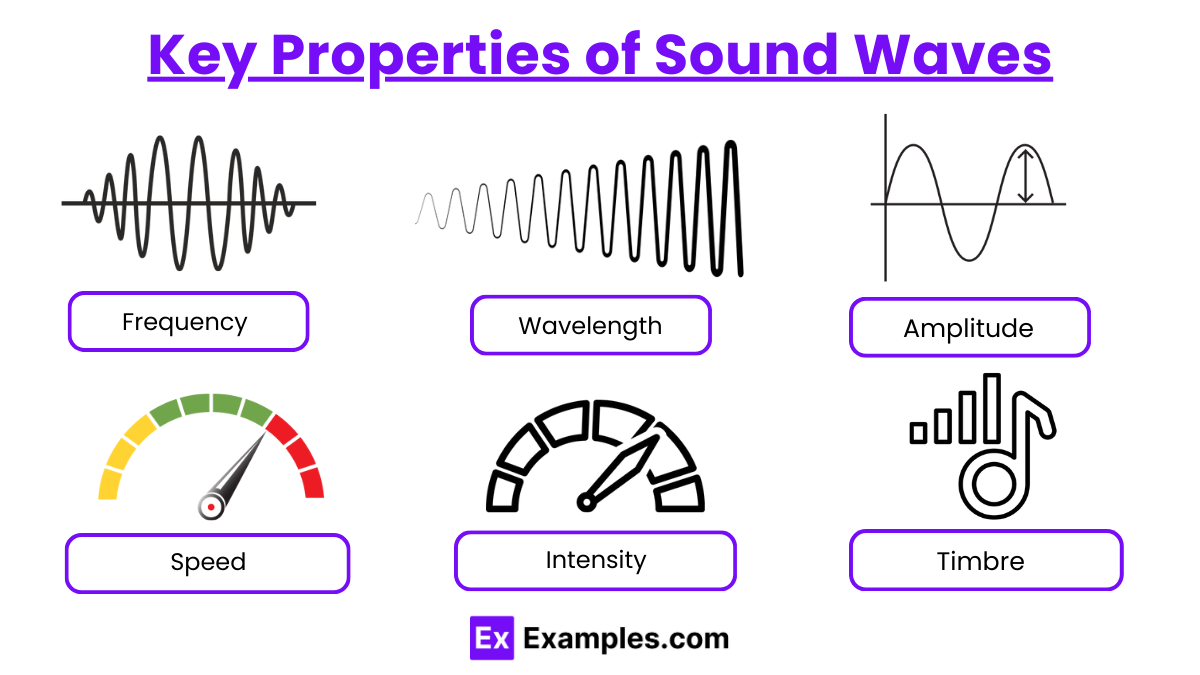
Sound waves, being mechanical waves, exhibit several key properties that determine how they interact with the environment and how we perceive them. These properties include frequency, wavelength, amplitude, speed, and intensity. Each of these characteristics has a specific impact on the behavior and effects of sound waves.
1. Frequency
Frequency is one of the most crucial aspects of sound waves. It refers to the number of wave cycles (oscillations) that pass a given point per unit of time, typically measured in hertz (Hz). Frequency determines the pitch of a sound:
- High Frequency: Corresponds to a high pitch.
- Low Frequency: Corresponds to a low pitch.
2. Wavelength
Wavelength is the distance between consecutive corresponding points of the same phase on the wave, such as from crest to crest or trough to trough. It is inversely proportional to frequency, meaning high-frequency sounds have shorter wavelengths, and low-frequency sounds have longer wavelengths. Wavelength is crucial for understanding the propagation of sound through different media.
3. Amplitude
Amplitude refers to the maximum displacement of a point on a wave from its rest position. In sound waves, it relates to the wave’s energy and is perceived as loudness; higher amplitudes are louder. Amplitude affects how sound waves carry energy and, in terms of energy transmission, the intensity of the sound.
4. Speed
The speed of sound varies depending on the medium through which the sound is traveling. It is fastest in solids, slower in liquids, and slowest in gases due to differences in the density and elastic properties of these media. For example:
- In air at 20°C (68°F), the speed of sound is about 343 meters per second (1,125 feet/second).
- Sound travels faster in water (about 1,480 meters/second) and even faster in steel (about 5,960 meters/second).
5. Intensity
Intensity is the power carried by sound waves per unit area, typically measured in watts per square meter (W/m²). It is a measure of the energy density of sound waves and is directly proportional to the square of the amplitude. Intensity determines the sound’s loudness as perceived by the human ear and is subject to the inverse square law, where intensity decreases as the distance from the sound source increases.
6. Timbre
While not a wave property in the physical sense, timbre (or tone color) is critical in how sound is perceived. It is what allows us to distinguish between different sources of sound having the same pitch and loudness. Timbre is influenced by the harmonic content of the sound and the dynamic characteristics of how the sound is produced and decayed.
Principles of Wave Interference, Resonance, and Standing Waves
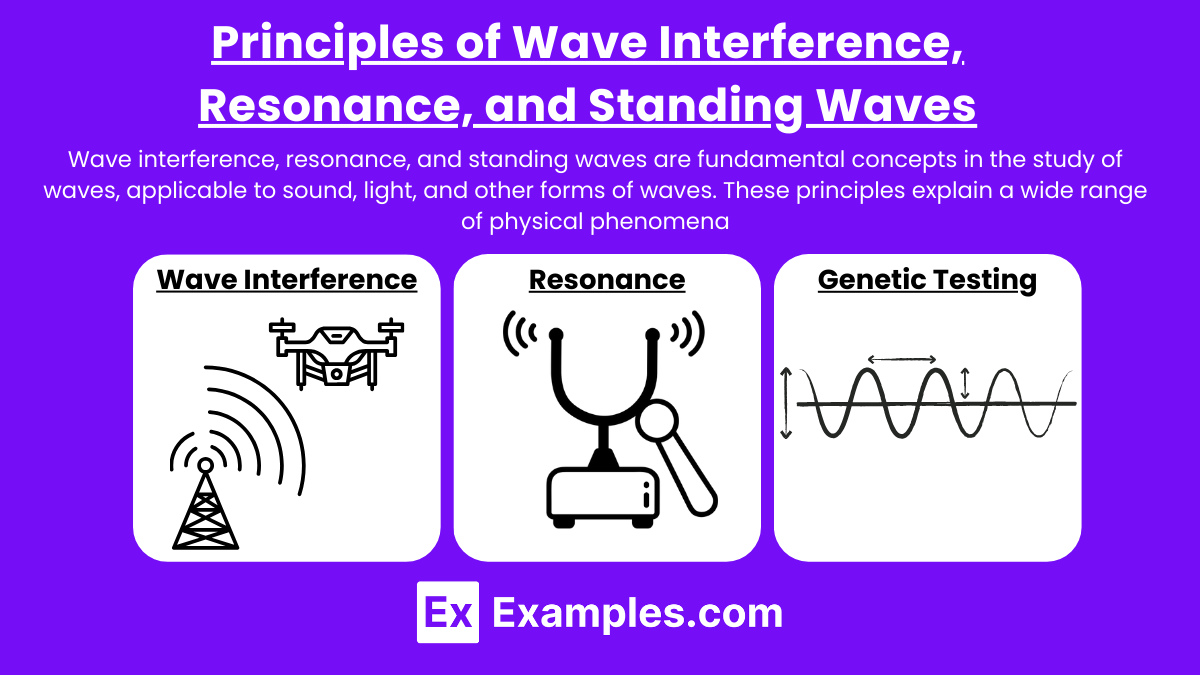
Wave interference, resonance, and standing waves are fundamental concepts in the study of waves, applicable to sound, light, and other forms of waves. These principles explain a wide range of physical phenomena, from the acoustics of musical instruments to the design of architectural spaces to enhance or suppress sound.
Wave Interference
Wave interference occurs when two or more waves meet while traveling through the same medium. The principle underlying wave interference is the superposition of wave functions:
- Constructive Interference: Occurs when the crests of one wave align with the crests of another, resulting in a wave of greater amplitude than either of the individual waves. This type of interference amplifies the wave effect.
- Destructive Interference: Occurs when the crest of one wave aligns with the trough of another, reducing or cancelling out the wave amplitude. This can lead to areas of significantly reduced wave intensity or even complete nullification.
Interference can be seen in many contexts, such as noise-canceling headphones which use destructive interference to reduce unwanted ambient sounds.
Resonance
Resonance is a phenomenon that occurs when a system is driven by another vibrating system or external force at a frequency that matches the system’s natural frequency. When this happens, the system oscillates with maximum amplitude.
- Examples:
- Musical Instruments: Strings on a guitar resonate at specific frequencies to produce sound. Wind instruments like flutes or trumpets resonate at particular harmonics depending on how the air column is vibrated.
- Structural Engineering: Buildings and bridges must be designed to avoid resonance with wind or earthquake vibrations, which could amplify forces and cause structural failure.
Standing Waves
Standing waves are a particular type of wave that appears to be stationary, meaning it does not seem to move through the medium. Instead, it oscillates in place. Standing waves occur due to the interference of two waves traveling in opposite directions with the same frequency and amplitude.
- Characteristics:
- Nodes: Points of zero amplitude that remain stationary.
- Antinodes: Points of maximum amplitude located midway between nodes.
The Doppler Effect and Its Applications
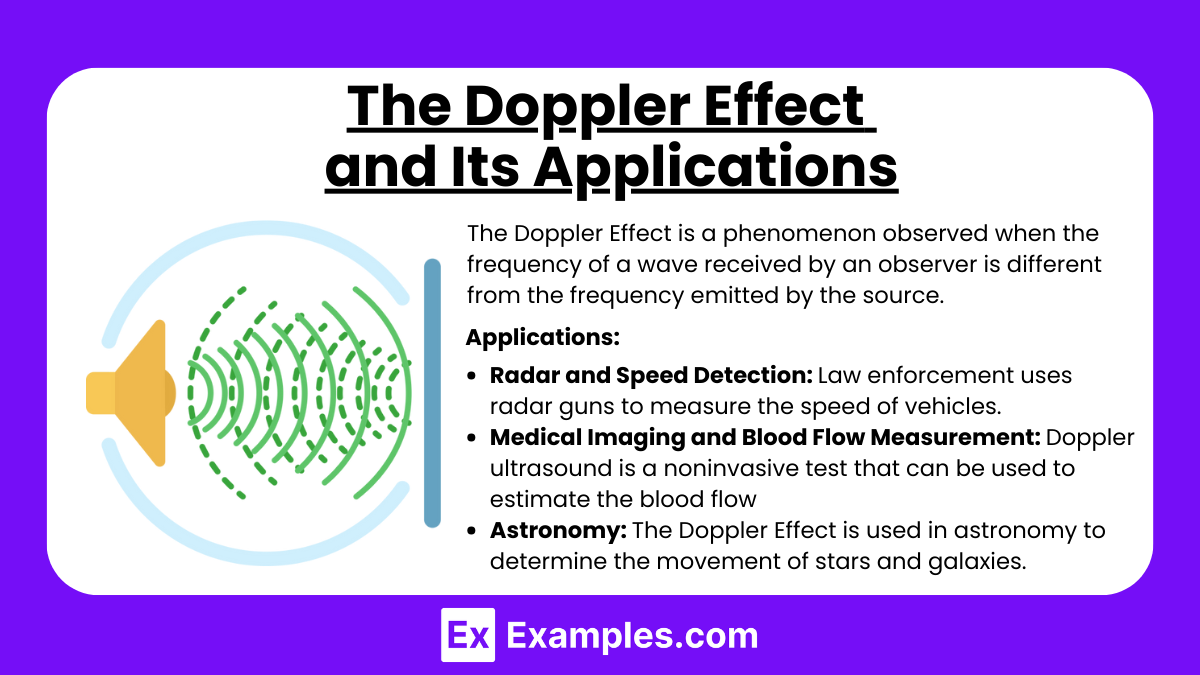
The Doppler Effect is a phenomenon observed when the frequency of a wave received by an observer is different from the frequency emitted by the source. This occurs when the source of the wave and the observer are moving relative to each other. The effect is named after Christian Doppler, who first proposed it in 1842. It applies to all types of waves, including sound, light, and electromagnetic waves.
Principles of the Doppler Effect
- Approaching Source: If the source of the waves is moving toward the observer, the wave fronts are compressed, leading to a higher frequency or pitch than when the source is stationary. This is perceived as an increase in pitch for sound waves.
- Receding Source: Conversely, if the source is moving away from the observer, the wave fronts are stretched, resulting in a lower frequency or pitch. This is perceived as a decrease in pitch for sound waves.
The formula to calculate the observed frequency (f′) is given by: f′=(v±vo/v∓vs)f
where:
- v is the speed of the wave in the medium,
- vo is the speed of the observer relative to the medium (positive if moving towards the source),
- vs is the speed of the source relative to the medium (positive if moving away from the observer),
- f is the actual frequency emitted by the source.
Applications of the Doppler Effect
- Radar and Speed Detection:
- Law enforcement uses radar guns to measure the speed of vehicles. The gun emits a radio wave that reflects off a moving vehicle; changes in the frequency of the reflected wave are used to calculate the vehicle’s speed.
- Similarly, Doppler radar is used in meteorology to detect weather patterns, such as the speed and direction of moving storm systems, by observing changes in the frequency of the returned signals.
- Medical Imaging and Blood Flow Measurement:
- Doppler ultrasound is a noninvasive test that can be used to estimate the blood flow through blood vessels by bouncing high-frequency sound waves (ultrasound) off circulating red blood cells. This is useful for diagnosing conditions like blood clots, heart valve defects, and arterial blockages.
- Astronomy:
- The Doppler Effect is used in astronomy to determine the movement of stars and galaxies relative to Earth. The shift in frequency of light from celestial objects allows astronomers to calculate whether these objects are moving toward or away from Earth, and at what speed.
- This application also extends to the study of exoplanets through Doppler spectroscopy, where the tiny wobbles in a star’s motion caused by the gravitational pull of an orbiting planet can be detected.
- Satellite Communication:
- In satellite communications, adjustments must be made for the Doppler Effect because the relative motion between the satellite and ground stations affects the frequency of the signals.
- Animal Echolocation:
- Animals like bats and dolphins use the Doppler Effect to navigate and hunt for prey through echolocation. They emit calls out to the environment and listen to the echoes of these calls that return from various objects near them.
Examples
Example 1: Musical Instruments
Different musical instruments produce sound through various methods, such as vibrating strings, air columns, or membranes. For example, a guitar produces sound when its strings vibrate after being plucked, creating longitudinal waves in the air. The frequency of the vibrations determines the pitch of the sound, while the body of the guitar amplifies the sound, showcasing the interplay between sound production and resonance.
Example 2: Echoes
An echo is a reflection of sound that occurs when sound waves bounce off surfaces and return to the listener. This phenomenon is commonly experienced in large, empty spaces, such as canyons or auditoriums. For example, if someone shouts in a canyon, the sound waves travel until they hit the canyon walls and reflect back, creating a delayed repetition of the original sound. This illustrates how sound waves can travel long distances and interact with their environment.
Example 3: Sonar Technology
Sonar (Sound Navigation and Ranging) uses sound waves to detect objects underwater. It is widely used in submarines and ships for navigation and mapping the ocean floor. Sonar systems emit sound pulses that travel through water, reflecting off objects like fish or underwater terrain. By measuring the time it takes for the sound waves to return, the distance to the object can be determined, showcasing the practical application of sound in technology.
Example 4: Speech and Communication
Human speech is a complex form of sound production that involves the vibration of vocal cords and the modulation of airflow. When we speak, our vocal cords create sound waves that travel through the air to reach listeners. The characteristics of these sound waves—such as pitch, volume, and tone—allow us to convey different emotions and meanings, illustrating the essential role of sound in communication.
Example 5: Acoustic Design in Architecture
The design of concert halls and auditoriums takes sound into account to enhance auditory experiences. Architects use materials and structural shapes to control how sound waves travel within the space, ensuring clarity and balance of sound. For instance, curved surfaces may be employed to direct sound waves towards the audience, while sound-absorbing materials help reduce echoes and background noise. This example highlights the importance of sound in architectural design and how it affects our perception of performance and speech.
Practice Question
Question 1
What is the primary medium through which sound travels the fastest?
A) Air
B) Water
C) Steel
D) Vacuum
Correct Answer: C) Steel.
Explanation: Sound travels fastest in solids compared to liquids and gases because the molecules in solids are packed closely together, allowing sound waves to transmit vibrations more efficiently. In air, sound travels slowly due to the larger distances between molecules, while in water, it travels faster than in air but still slower than in steel. Sound cannot travel in a vacuum since there are no molecules to carry the sound waves, making option D incorrect.
Question 2
What characteristic of sound is primarily determined by its frequency?
A) Loudness
B) Pitch
C) Timbre
D) Duration
Correct Answer: B) Pitch.
Explanation: The pitch of a sound is determined by its frequency, which is the number of vibrations or cycles per second. Higher frequency sounds correspond to higher pitches, while lower frequencies correspond to lower pitches. Loudness is related to the amplitude of the sound wave, timbre refers to the quality or color of the sound that distinguishes different types of sound sources, and duration pertains to how long a sound lasts. Therefore, option B is correct.
Question 3
Which of the following statements is true about sound waves?
A) Sound waves can travel through a vacuum.
B) Sound waves are longitudinal waves.
C) Sound waves have no effect on matter.
D) Sound waves travel faster in gas than in solids.
Correct Answer: B) Sound waves are longitudinal waves.
Explanation: Sound waves are classified as longitudinal waves because the particles of the medium through which they travel vibrate parallel to the direction of the wave propagation. This results in compressions and rarefactions within the medium. Option A is incorrect because sound requires a medium (solid, liquid, or gas) to propagate and cannot travel through a vacuum. Option C is also incorrect; sound waves cause particles in the medium to vibrate, so they do affect matter. Lastly, option D is false; sound travels faster in solids than in gases due to the closer arrangement of molecules in solids, which facilitates quicker transmission of sound.