Stoichiometry is fundamental to chemistry, focusing on the quantitative relationships between reactants and products in chemical reactions. On the MCAT, you’ll explore mole concepts, balancing chemical equations, and limiting reagents. Understanding theoretical yield, percent yield, and the application of stoichiometric coefficients is essential, as these concepts are key to mastering general chemistry and chemical reactions sections of the exam.
Learning Objectives
In studying “Stoichiometry” for the MCAT, you should learn to interpret balanced chemical equations and understand the mole concept in relation to chemical reactions. Develop proficiency in calculating the amounts of reactants and products involved using mole ratios. Analyze limiting reactants and determine how they affect the yield of a chemical reaction. Understand how to calculate theoretical, actual, and percent yields. Practice converting between mass, moles, and molecules to solve stoichiometric problems efficiently. Additionally, evaluate stoichiometry in real-world contexts, such as biochemical pathways and metabolism, to prepare for MCAT questions that integrate chemistry with biology.
Converting Between Mass, Moles, and Molecules
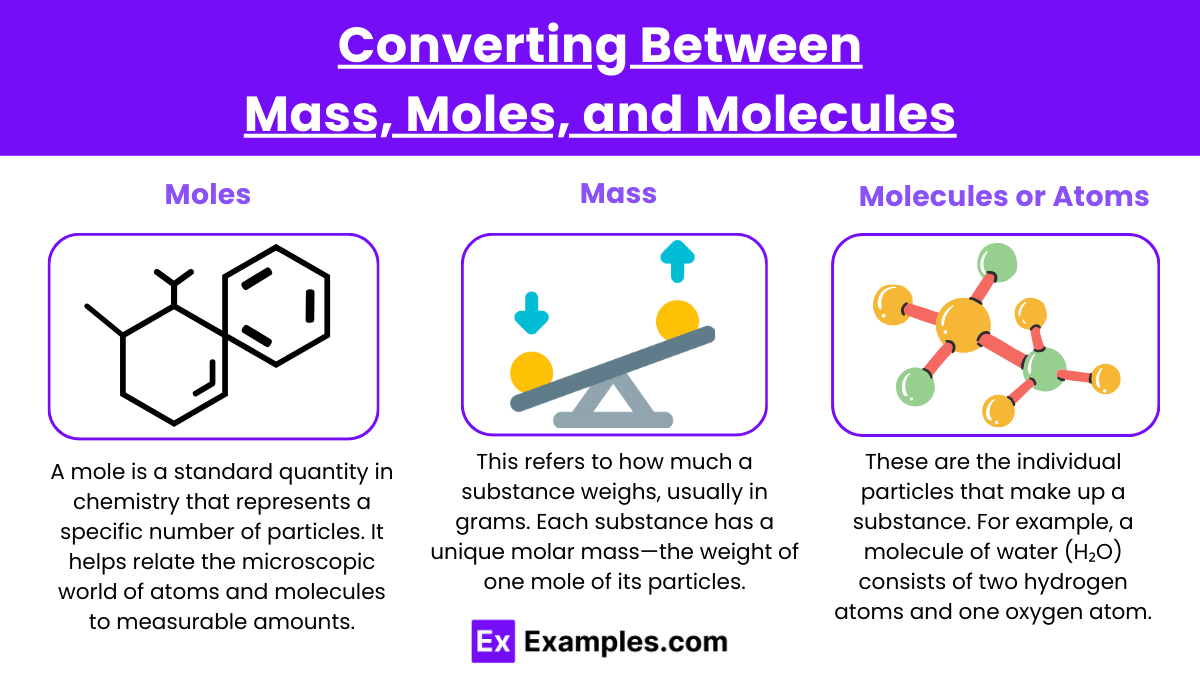
Understanding how to convert between mass, moles, and molecules is essential in chemistry, as it allows precise calculations for chemical reactions and substance quantities. Here’s an overview of the process and its practical applications.
Moles: A mole is a standard quantity in chemistry that represents a specific number of particles—about 6.022×1023 (Avogadro’s number). It helps relate the microscopic world of atoms and molecules to measurable amounts.
Mass: This refers to how much a substance weighs, usually in grams. Each substance has a unique molar mass—the weight of one mole of its particles—expressed in grams per mole.
Molecules or Atoms: These are the individual particles that make up a substance. For example, a molecule of water (H₂O) consists of two hydrogen atoms and one oxygen atom.
Steps for Conversion
- Mass to Moles: Divide the given mass by the molar mass of the substance to determine how many moles are present.
- Moles to Mass: Multiply the number of moles by the molar mass to find the corresponding weight.
- Moles to Molecules/Atoms: Use Avogadro’s number to convert moles into individual particles. One mole contains approximately 6.022×10236.022 \times 10^{23}6.022×1023 molecules or atoms.
- Molecules/Atoms to Moles: Divide the number of particles by Avogadro’s number to find the amount in moles.
Example Scenarios
- Mass to Moles: Knowing the weight of a substance helps you determine the number of moles involved in a chemical reaction. For instance, 18 grams of water equals one mole, which helps in calculating reactant amounts.
- Moles to Molecules: If you have two moles of carbon dioxide, multiplying it by Avogadro’s number gives the total number of CO₂ molecules involved, helping in reaction planning.
- Molecules to Mass: If you know the number of water molecules, you can determine how much they weigh in grams by first converting them to moles and then to mass.
Interpreting Balanced Chemical Equations and the Mole Concept
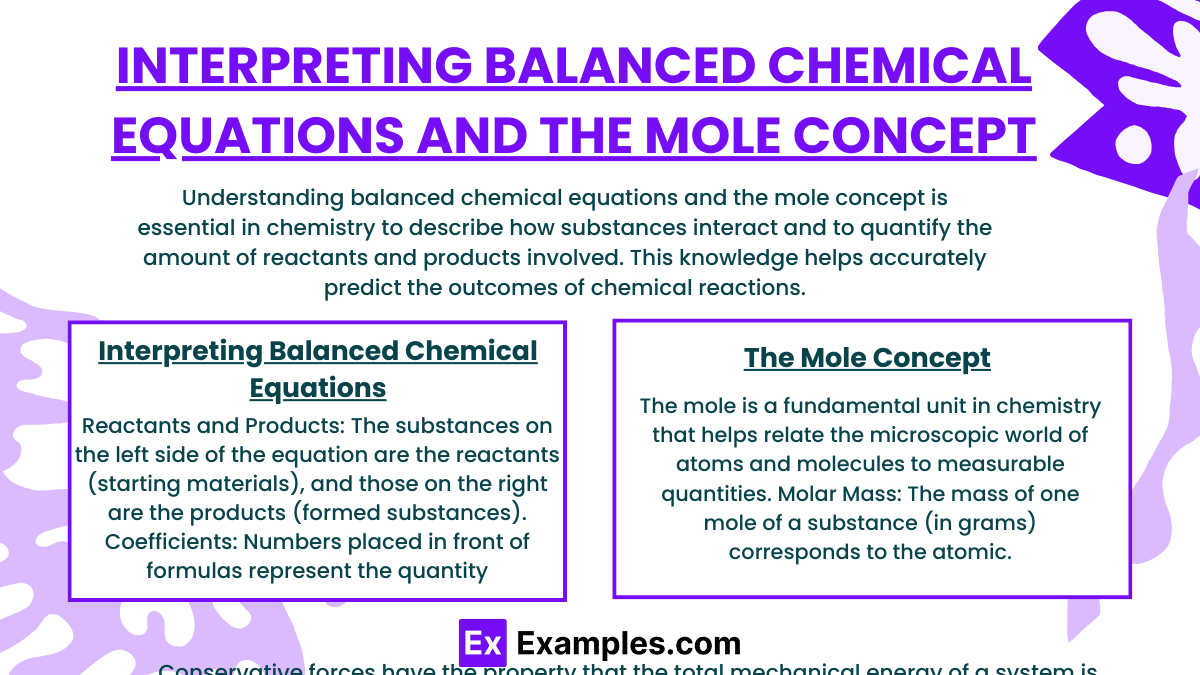
Understanding balanced chemical equations and the mole concept is essential in chemistry to describe how substances interact and to quantify the amount of reactants and products involved. This knowledge helps accurately predict the outcomes of chemical reactions.
Interpreting Balanced Chemical Equations
A balanced chemical equation provides the precise relationship between the number of particles (atoms, molecules, or ions) of each substance involved in a chemical reaction. The equation ensures that the law of conservation of mass is upheld, meaning the number of atoms of each element is the same on both sides of the equation.
- Reactants and Products: The substances on the left side of the equation are the reactants (starting materials), and those on the right are the products (formed substances).
- Coefficients: Numbers placed in front of formulas represent the quantity (in moles) of each substance involved in the reaction. These coefficients ensure that the equation is balanced.
- States of Matter: Symbols like (s), (l), (g), and (aq) indicate the state of each substance: solid, liquid, gas, or aqueous (dissolved in water).
Example: In the combustion of methane: CH4+2O2 → CO2+2H2O
This balanced equation tells us that one molecule of methane reacts with two molecules of oxygen to produce one molecule of carbon dioxide and two molecules of water.
The Mole Concept
The mole is a fundamental unit in chemistry that helps relate the microscopic world of atoms and molecules to measurable quantities.
- One mole contains 6.022×1023 particles (Avogadro’s number), which can represent atoms, molecules, or ions, depending on the context.
- Molar Mass: The mass of one mole of a substance (in grams) corresponds to the atomic or molecular mass from the periodic table. For example, one mole of water (H₂O) weighs 18 grams.
Connecting Chemical Equations to the Mole Concept
- Stoichiometry: Using the coefficients in a balanced chemical equation, we can determine how many moles of reactants are needed to produce a given amount of product.
- Mole Ratios: The ratios of reactants and products are crucial for calculating quantities. In the methane example, the equation tells us that for every 1 mole of methane, 2 moles of oxygen are required, and 1 mole of carbon dioxide and 2 moles of water are produced.
Analyzing Limiting Reactants and Their Effect on Yield
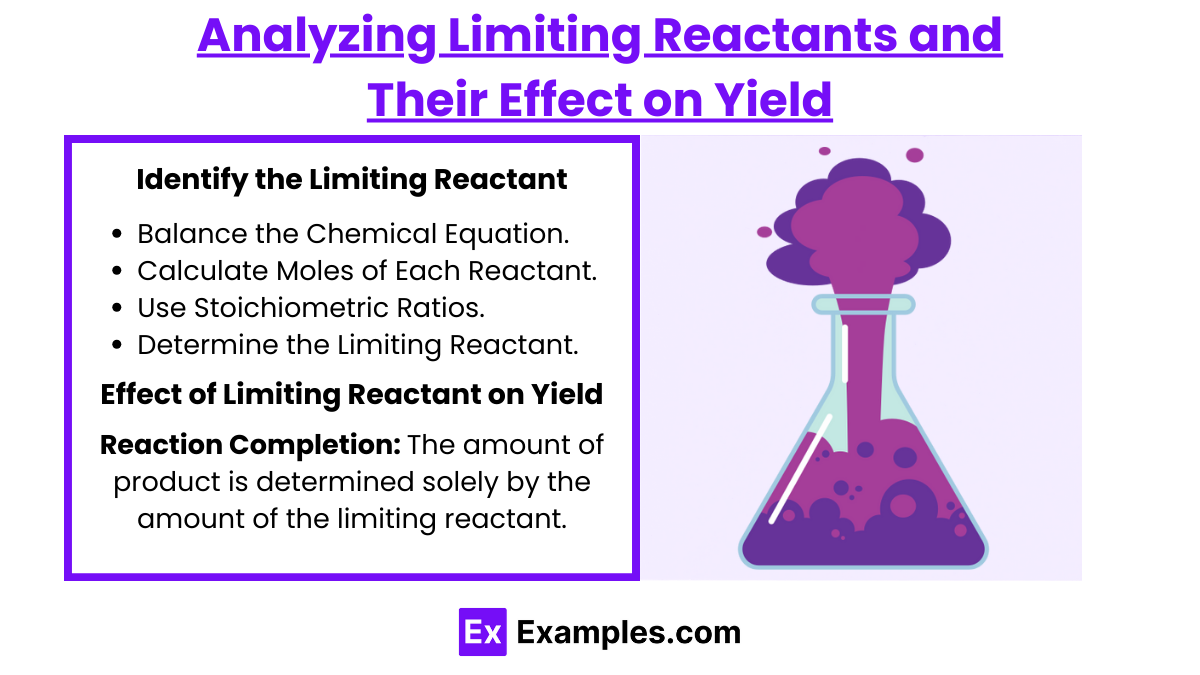
In a chemical reaction, the limiting reactant (or limiting reagent) is the substance that is completely consumed first, limiting the amount of product that can be formed. Understanding how to identify the limiting reactant is essential for optimizing chemical reactions and accurately predicting product yields.
Key Concepts
- Limiting Reactant:
- The reactant that runs out first, preventing the formation of more product.
- Once the limiting reactant is used up, the reaction stops, even if other reactants are still available.
- Excess Reactant: The reactant(s) that remain after the limiting reactant is consumed. These are left over at the end of the reaction.
- Theoretical Yield: The maximum amount of product that can be formed based on the complete consumption of the limiting reactant. It is calculated using stoichiometry from the balanced chemical equation.
- Actual Yield: The amount of product actually obtained from the reaction. It is often lower than the theoretical yield due to side reactions, losses, or incomplete reactions.
- Percent Yield: A measure of the efficiency of a reaction, calculated as: Percent Yield = (Actual Yield/Theoretical Yield) × 100
Steps to Identify the Limiting Reactant
- Balance the Chemical Equation: Ensure the equation is balanced, meaning the number of atoms of each element is the same on both sides of the equation.
- Calculate Moles of Each Reactant: Convert the mass of each reactant to moles using their molar masses.
- Use Stoichiometric Ratios: Compare the mole ratios from the balanced equation to determine which reactant will run out first.
- Determine the Limiting Reactant: The reactant that produces the smallest amount of product is the limiting reactant.
Example: Identifying the Limiting Reactant
Reaction: 2H2+O2 → 2H2
- If you start with 4 moles of hydrogen and 2 moles of oxygen:
- According to the equation, 2 moles of hydrogen react with 1 mole of oxygen to form water.
- With 4 moles of hydrogen, you would need 2 moles of oxygen to completely react.
Since both reactants are exactly in the required ratio, neither is limiting in this case, and both will be fully consumed.
Effect of Limiting Reactant on Yield
- Reaction Completion: The amount of product is determined solely by the amount of the limiting reactant. No matter how much excess reactant is available, it cannot increase the product yield.
- Efficiency and Waste Reduction: Identifying the limiting reactant helps in optimizing the amounts of reactants used, reducing waste and maximizing efficiency, which is especially important in industrial processes.
Evaluating Stoichiometry in Real-World Contexts
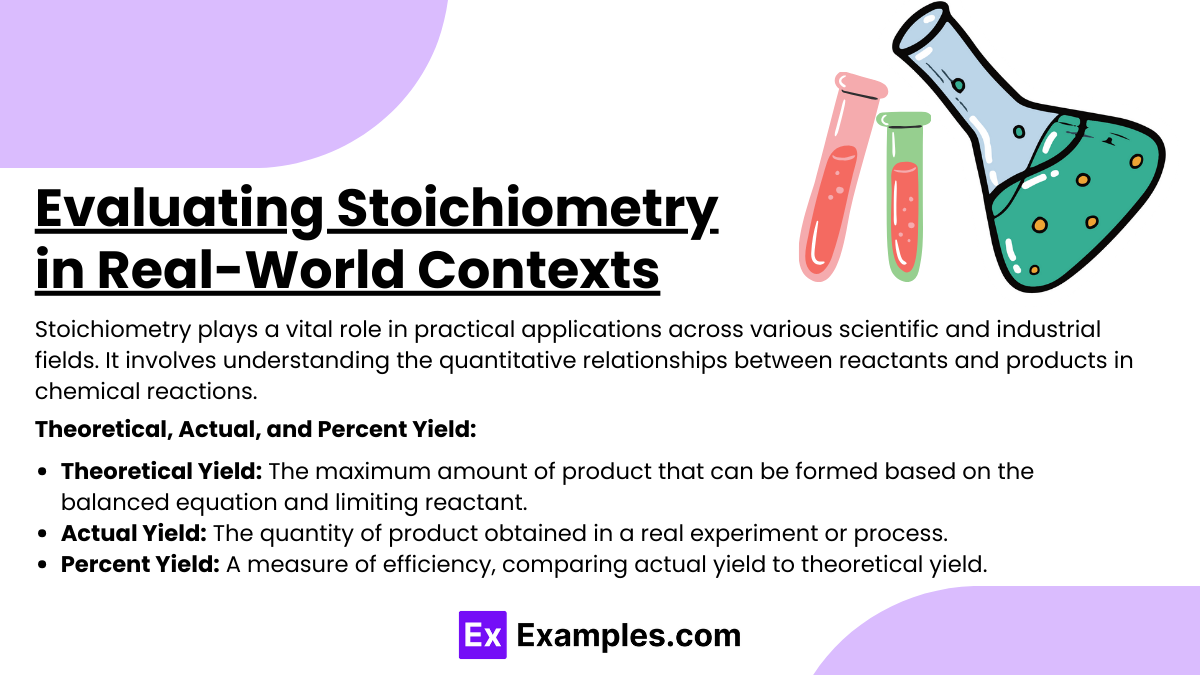
Stoichiometry plays a vital role in practical applications across various scientific and industrial fields. It involves understanding the quantitative relationships between reactants and products in chemical reactions, ensuring efficient use of materials and accurate predictions of outcomes.
Mole Ratios: Balanced chemical equations provide the mole ratios of reactants and products, which help predict the amounts required or formed during a reaction.
Limiting and Excess Reactants: Determining the limiting reactant ensures that the reaction proceeds efficiently without wasting materials. Excess reactants may be left over but do not affect the amount of product formed.
Theoretical, Actual, and Percent Yield:
- Theoretical Yield: The maximum amount of product that can be formed based on the balanced equation and limiting reactant.
- Actual Yield: The quantity of product obtained in a real experiment or process.
- Percent Yield: A measure of efficiency, comparing actual yield to theoretical yield.
Applications of Stoichiometry in Real-World Contexts
1. Chemical Manufacturing
- Optimization of Reactants: Stoichiometry ensures that precise amounts of chemicals are mixed to minimize waste and reduce costs in large-scale production (e.g., fertilizers, pharmaceuticals).
- Controlling By-products: It helps predict and limit unwanted by-products, improving the purity of the desired product and meeting environmental regulations.
2. Pharmaceutical Industry
- Drug Synthesis: Accurate stoichiometric calculations ensure that precise dosages are produced, avoiding excess waste or underproduction.
- Formulations: Stoichiometry is used to determine the correct proportions of active and inactive ingredients in medications to ensure efficacy and safety.
3. Environmental Science
- Air Pollution Control: Stoichiometry helps in reactions like scrubbing pollutants from exhaust gases. For instance, calculating the exact amount of reactant (like calcium hydroxide) required to neutralize sulfur dioxide emissions ensures efficiency and reduces environmental harm.
- Wastewater Treatment: Reactions used in treating wastewater rely on stoichiometry to predict how much chemical agent is needed to break down contaminants.
4. Food Chemistry and Nutrition
- Fermentation: In fermentation processes, such as brewing or baking, stoichiometry predicts the amount of sugar required to produce the desired amount of ethanol or carbon dioxide.
- Nutritional Formulas: Stoichiometry is applied to balance nutrients in supplements and fortified foods, ensuring correct dosages and preventing nutrient imbalance.
5. Energy Production
- Combustion Reactions: In power plants or engines, stoichiometry ensures the correct fuel-to-oxygen ratio, maximizing efficiency and reducing emissions.
- Battery Design: In electrochemical cells, stoichiometry predicts how much reactant is required to generate a specific amount of electrical energy.
Examples
Example 1: Combustion of Hydrocarbons
Stoichiometry plays a crucial role in determining the amounts of reactants and products in the combustion of hydrocarbons, such as propane (C3H8). The balanced equation for the complete combustion of propane is C3H8+5O2→3CO2+4H2O. Using stoichiometry, chemists can calculate how much oxygen is required to completely combust a given amount of propane, as well as the amounts of carbon dioxide and water produced.
Example 2: Synthesis of Ammonia
In the Haber process, nitrogen (N2) and hydrogen (H2) gases react to form ammonia (NH3). The balanced equation is N2+3H2→2NH3. Stoichiometric calculations allow chemists to determine how many moles of hydrogen are needed to react with a specific amount of nitrogen, ensuring optimal yields of ammonia in industrial settings.
Example 3: Titration Reactions
In a titration experiment, stoichiometry is used to determine the concentration of an unknown solution. For instance, when titrating hydrochloric acid (HCl) with sodium hydroxide (NaOH), the balanced equation is HCl+NaOH→NaCl+H2O. By knowing the concentration and volume of one reactant, stoichiometry helps calculate the concentration of the other, allowing for precise measurements in analytical chemistry.
Example 4: Precipitation Reactions
Stoichiometry is essential in predicting the formation of precipitates in reactions involving ionic compounds. For example, when solutions of silver nitrate (AgNO3) and sodium chloride (NaCl) are mixed, the reaction can be represented as AgNO3+NaCl→AgCl(s)+NaNO3. By applying stoichiometric principles, chemists can calculate the amounts of each reactant needed to produce a specific amount of precipitate, in this case, silver chloride (AgCl).
Example 5: Gas Reactions and Molar Volume
Stoichiometry is utilized in reactions involving gases, especially when applying the ideal gas law. For example, in the reaction between hydrogen gas (H2) and oxygen gas (O2) to produce water (H2O), the balanced equation is 2H2+O2→2H2O. Stoichiometric calculations can determine the volumes of gases consumed or produced at standard temperature and pressure (STP), demonstrating how gas behavior and stoichiometry are interconnected in chemical reactions.
Practice Questions
Question 1
What is the primary purpose of stoichiometry in chemical reactions?
A) To measure the temperature of reactants
B) To determine the quantities of reactants and products involved in a reaction
C) To analyze the color changes during a reaction
D) To calculate the speed of the reaction
Correct Answer: B) To determine the quantities of reactants and products involved in a reaction.
Explanation: Stoichiometry is the branch of chemistry that deals with the relationships between the quantities of reactants and products in a chemical reaction. It allows chemists to predict how much of each substance is required or produced, enabling efficient planning and execution of reactions. Options A, C, and D are irrelevant to stoichiometry, as they do not relate to the quantitative relationships in chemical reactions.
Question 2
In a balanced chemical equation, what do the coefficients represent?
A) The temperature of the reaction
B) The number of moles of each substance involved
C) The time taken for the reaction to complete
D) The energy change during the reaction
Correct Answer: B) The number of moles of each substance involved.
Explanation: The coefficients in a balanced chemical equation indicate the relative number of moles of each substance that participate in the reaction. For example, in the reaction 2H2+O2→2H2O, the coefficient “2” before H2 means that two moles of hydrogen react with one mole of oxygen to produce two moles of water. This stoichiometric relationship is essential for accurately predicting how reactants convert to products. Options A, C, and D do not accurately describe the role of coefficients in stoichiometry.
Question 3
What does the term “limiting reactant” refer to in a chemical reaction?
A) The reactant that is present in excess
B) The reactant that is completely consumed first
C) The reactant that determines the temperature of the reaction
D) The reactant that is not involved in the reaction
Correct Answer: B) The reactant that is completely consumed first.
Explanation: The limiting reactant is the substance that is completely consumed when the reaction goes to completion. It limits the amount of product that can be formed, as no further reactions can occur without it. Identifying the limiting reactant is crucial for calculating the theoretical yield of products in a chemical reaction. Option A describes the excess reactant, while options C and D are unrelated to the concept of limiting reactants.