Raman Scattering
Raman Scattering, also known as Raman Effect, is a phenomenon in physics where light scatters inelastically off molecules, causing the scattered light to shift in energy and wavelength. This scattering occurs when photons interact with molecular vibrations, rotations, or other low-frequency modes in a substance. The change in energy and wavelength of the photons provides insights into the molecular structure and properties of the material. This physical process is governed by the fundamental laws of physics.
What is Raman Scattering?
What is Raman Spectrometer?
A Raman Spectrometer is an analytical instrument used to observe Raman Scattering and measure the Raman spectra of various materials. It primarily functions by shining a monochromatic light source, such as a laser, onto a sample. The light interacts with the molecules in the sample, resulting in the energy of the light being shifted due to vibrational, rotational, and other low-frequency modes within the molecules.
What is Raman Spectra?
Raman Spectra represent the specific pattern of scattered light produced when light interacts with the molecules of a substance through Raman Scattering. This spectrum displays the intensity of scattered light as a function of its frequency shift relative to the incident light.
When a light source, typically a laser, illuminates a sample, most photons scatter elastically without a change in energy, known as Rayleigh scattering. However, a small fraction of these photons undergo inelastic scattering, gaining or losing energy by interacting with the molecular vibrations of the sample. This change in energy results in a shift in the wavelength of the scattered light, which is captured in the Raman spectra.
Degrees of Freedom
Degrees of freedom in physics refer to the independent parameters that define the state of a physical system. These parameters determine the number of independent movements a system can make, encompassing both translational and rotational movements.
In the context of mechanics, degrees of freedom are the individual motions (translations and rotations) that a physical body can undergo in three-dimensional space. For example, a free particle moving in space has three translational degrees of freedom, corresponding to movement along the X, Y, and Z axes. If the particle can also rotate, it may have additional rotational degrees of freedom.
What is Raman Spectroscopy?
Raman Spectroscopy is a spectroscope technique that measures the Raman spectra of molecules to provide insights into their chemical structures, compositions, and interactions. The technique involves illuminating a sample with a laser light, which interacts with the molecular vibrations, phonons, or other excitations in the sample, leading to the scattering of the light at different energies.
Raman spectroscopy is distinguished by its ability to provide detailed information about molecular vibrations through the interaction of light with matter. Central to understanding Raman spectroscopy are the concepts of Stokes and Anti-Stokes scattering. These phenomena describe how photons scatter when they interact with molecules, leading to shifts in energy that are characteristic of the molecular structure of the sample.
Stokes scattering occurs when photons in the incident light lose energy to the sample during interaction. This energy loss corresponds to an increase in the vibrational energy of the molecules in the sample.
Anti-Stokes scattering occurs when photons in the incident light gain energy from the sample. This happens when a molecule in an excited vibrational state transfers energy to the photon.
Principle of Raman Spectroscopy
Raman Spectroscopy operates on the principle of Raman scattering, where monochromatic light, typically from a laser, interacts with molecular vibrations, rotations, or other forms of low-frequency modes within a material. As the laser light illuminates the sample, most of the light scatters elastically (Rayleigh scattering), which does not alter the energy of the photons. However, a small fraction of the light scatters inelastically (Raman scattering). Resulting in a shift in energy relative to the incident photons.
This shift in energy provides vital information about the molecular structure of the sample. It occurs because the photons either lose or gain energy by interacting with the vibrational energy states of the molecules in the sample. The amount of energy shift and its direction (higher or lower energy) indicate the specific vibrational modes of the molecules. Which are characteristic of their chemical bonds and molecular interactions.
Types of Raman Spectroscopy
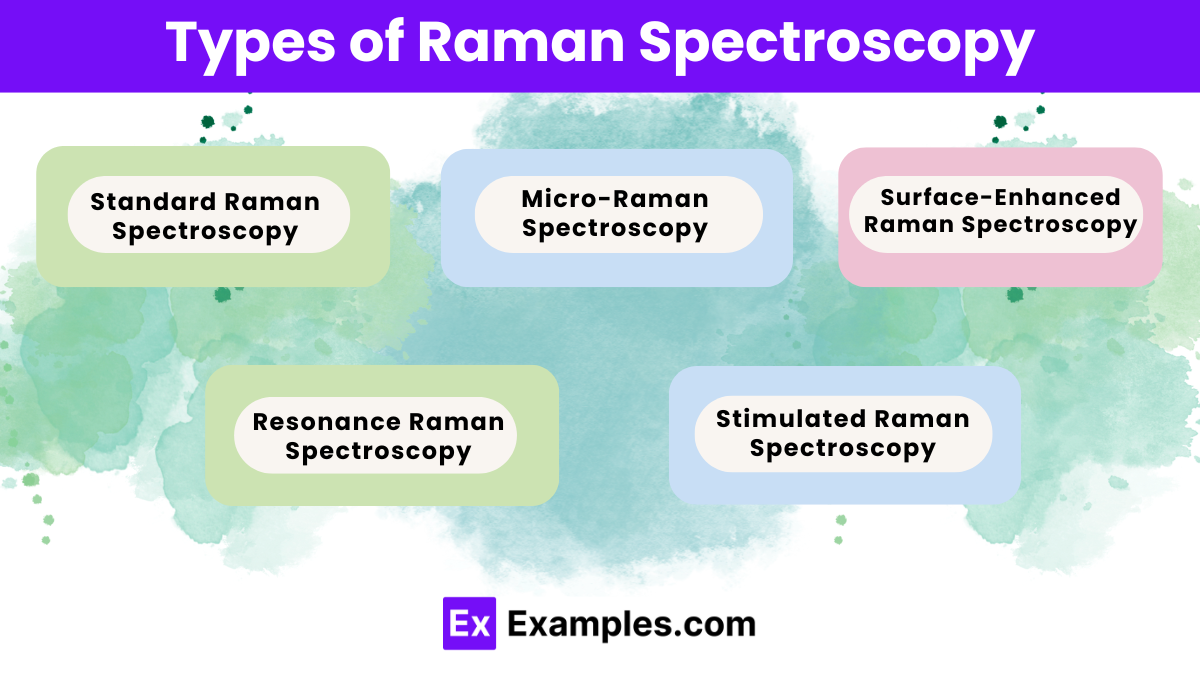
Raman Spectroscopy comes in different types, each suited for specific applications:
Standard Raman Spectroscopy:
- Most common type.
- Uses a visible light laser to analyze the sample.
Micro-Raman Spectroscopy:
- Uses a microscope to focus the laser on a small part of the sample.
- Good for studying tiny areas.
Surface-Enhanced Raman Spectroscopy (SERS):
- Increases the Raman signal using a rough metal surface.
- Useful for detecting low concentrations of chemicals.
Resonance Raman Spectroscopy:
- Enhances Raman signals by using laser light that matches the color absorbed by the sample.
- Good for studying colored molecules.
Stimulated Raman Spectroscopy:
- Uses laser pulses to increase the intensity of the Raman effect.
- Allows for faster and more sensitive measurements.
Uses of Raman Scattering
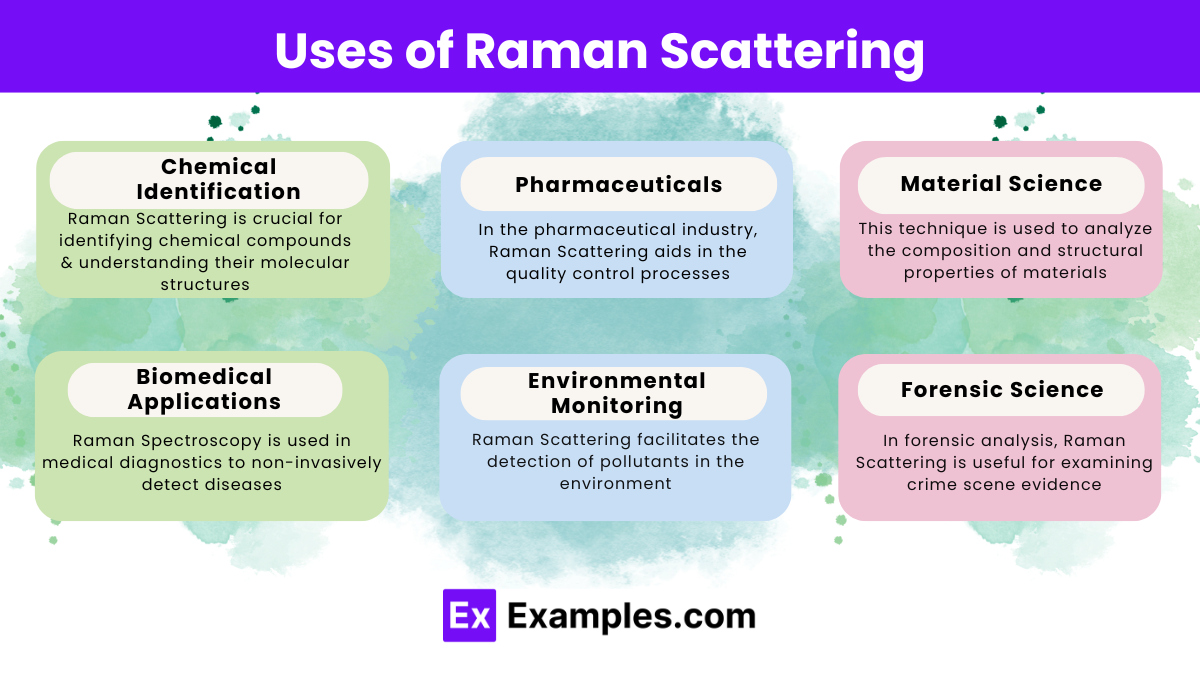
- Chemical Identification: Raman Scattering is crucial for identifying chemical compounds and understanding their molecular structures. It provides a molecular fingerprint that is unique to each substance. Making it invaluable in chemistry labs for substance identification and purity checks.
- Pharmaceuticals: In the pharmaceutical industry, Raman Scattering aids in the quality control processes by determining the composition and confirming the structure of pharmaceutical ingredients. It ensures that drugs are pure and effective.
- Material Science: This technique is used to analyze the composition and structural properties of materials, including ceramics, polymers, and semiconductors. Raman Scattering helps in the development of new materials with desired properties and improvements.
- Biomedical Applications: Raman Spectroscopy is used in medical diagnostics to non-invasively detect diseases by analyzing tissue samples. It can identify biochemical changes in cells that may indicate disease states.
- Environmental Monitoring: Raman Scattering facilitates the detection of pollutants in the environment. It can identify hazardous substances in water, air, and soil, aiding in environmental protection and regulation compliance.
- Forensic Science: In forensic analysis, Raman Scattering is useful for examining crime scene evidence. Such as drug residues, fibers, or explosive materials. Without damaging the original samples.
Examples for Raman Scattering
Raman Scattering is a powerful tool utilized across numerous disciplines. Here are some practical examples demonstrate its widespread applications:
- Chemical Analysis: Scientists use Raman Spectroscopy to determine the chemical composition of unknown substances in a lab. For instance, it can different between similar-looking white powders in forensic analysis by identify their molecular structures.
- Pharmaceutical Quality Control: Pharmaceutical companies apply Raman Spectroscopy to check the quality of raw materials and finished products. It can rapidly identify contaminants or ensure that the correct crystal form of a compound is present in the final product.
- Studying Stress in Materials: Engineers use Raman Spectroscopy to assess stress and strain in materials like semiconductors and ceramics. This technique helps in understanding how materials deform under stress, which is crucial for structural analysis.
- Biomedical Diagnostics: In biomedical research, Raman Spectroscopy helps diagnose diseases by detecting molecular changes in tissues. For example, it can identify changes in bone composition or detect early signs of cancers based on the molecular signatures in cells.
- Environmental Monitoring: Raman Spectroscopy is employed to detect and quantify pollutants in water or air. It enables environmental scientists to identify toxic substances such as PCBs in water samples without needing extensive sample preparation.
- Art Restoration and Analysis: Conservators use Raman Spectroscopy to help restore artwork. It can identify the pigments and materials used in historical paintings, aiding in the decision-making process for restore techniques that will not damage the original works.
FAQ’s
Who discovered Raman Scattering?
Indian physicist C.V. Raman discovered Raman Scattering in 1928, earning him the Nobel Prize in Physics in 1930.
How does Raman Scattering occur?
It occurs when incident photons interact with molecules, inducing energy changes and resulting in scatter light with different frequencies.
What is the significance of Raman Scattering?
It provides valuable information about molecular vibrations, structures, and interactions, aiding in various fields like chemistry, materials science, and biomedical research.
How is Raman Scattering different from fluorescence?
Raman Scattering involves energy-level changes without emission of light, while fluorescence involves emission of light after absorb photons.
What instruments are used for Raman Spectroscopy?
Raman spectrometers, equipped with lasers, detectors, and spectrometers, are used to analyze scattered light and generate Raman spectra.
What is resonance Raman spectroscopy?
It’s a variant of Raman spectroscopy where the excitation wavelength matches the electronic transition energy of the molecule, enhance Raman signals.
How does Raman Scattering contribute to materials science?
It characterizes materials’ chemical compositions, structures, and phases, facilitate research in nanotechnology, polymers, and semiconductors.
Can Raman Scattering detect subtle molecular changes?
Yes, it’s sensitive to subtle changes in molecular vibrations, making it valuable for detecting structural variations and chemical interactions.
What factors affect Raman Scattering intensity?
Factors include excitation wavelength, sample concentration, laser power, and scattering angle, influence signal strength and spectral quality.
What challenges are associated with Raman Scattering?
Challenges include fluorescence interference, low signal-to-noise ratios, and complex spectral interpretation, require advanced instrumentation and data analysis techniques.